MXene-Based Elastomer Mimetic Stretchable Sensors: Design, Properties, and Applications
- PMID: 38411801
- PMCID: PMC10899156
- DOI: 10.1007/s40820-024-01349-w
MXene-Based Elastomer Mimetic Stretchable Sensors: Design, Properties, and Applications
Abstract
Flexible sensors based on MXene-polymer composites are highly prospective for next-generation wearable electronics used in human-machine interfaces. One of the motivating factors behind the progress of flexible sensors is the steady arrival of new conductive materials. MXenes, a new family of 2D nanomaterials, have been drawing attention since the last decade due to their high electronic conductivity, processability, mechanical robustness and chemical tunability. In this review, we encompass the fabrication of MXene-based polymeric nanocomposites, their structure-property relationship, and applications in the flexible sensor domain. Moreover, our discussion is not only limited to sensor design, their mechanism, and various modes of sensing platform, but also their future perspective and market throughout the world. With our article, we intend to fortify the bond between flexible matrices and MXenes thus promoting the swift advancement of flexible MXene-sensors for wearable technologies.
Keywords: 2D nanomaterials; Applications; Flexible sensor; MXene; Wearable and conductive.
© 2024. The Author(s).
Conflict of interest statement
The authors declare no interest conflict. They have no known competing financial interests or personal relationships that could have appeared to influence the work reported in this paper.
Figures
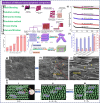
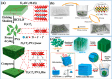
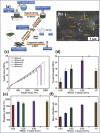
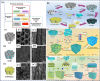
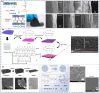
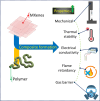
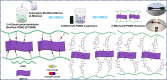
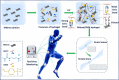
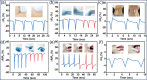
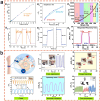
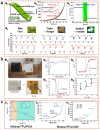
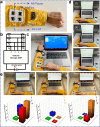
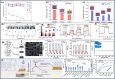
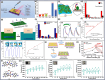
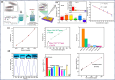
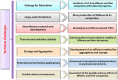
Similar articles
-
MXene-Based Textile Sensors for Wearable Applications.ACS Sens. 2022 Apr 22;7(4):929-950. doi: 10.1021/acssensors.2c00097. Epub 2022 Mar 24. ACS Sens. 2022. PMID: 35322661 Review.
-
Advancements in MXene Composite Materials for Wearable Sensors: A Review.Sensors (Basel). 2024 Jun 24;24(13):4092. doi: 10.3390/s24134092. Sensors (Basel). 2024. PMID: 39000870 Free PMC article. Review.
-
Recent Progress in MXene Hydrogel for Wearable Electronics.Biosensors (Basel). 2023 Apr 22;13(5):495. doi: 10.3390/bios13050495. Biosensors (Basel). 2023. PMID: 37232856 Free PMC article. Review.
-
MXene Fibers for Flexible and Wearable Electronics: Recent Progress and Future Perspectives.Chem Asian J. 2023 Aug 15;18(16):e202300474. doi: 10.1002/asia.202300474. Epub 2023 Jul 24. Chem Asian J. 2023. PMID: 37427996 Review.
-
Recent Advances in Flexible Pressure Sensors Based on MXene Materials.Adv Mater. 2024 Jun;36(24):e2312761. doi: 10.1002/adma.202312761. Epub 2024 Mar 13. Adv Mater. 2024. PMID: 38380773 Review.
Cited by
-
Flexible Sensors Based on Conductive Polymer Composites.Sensors (Basel). 2024 Jul 18;24(14):4664. doi: 10.3390/s24144664. Sensors (Basel). 2024. PMID: 39066060 Free PMC article. Review.
-
Flexible Strain Sensors with Ultra-High Sensitivity and Wide Range Enabled by Crack-Modulated Electrical Pathways.Nanomicro Lett. 2024 Nov 18;17(1):64. doi: 10.1007/s40820-024-01571-6. Nanomicro Lett. 2024. PMID: 39551898 Free PMC article.
References
-
- S. He, X. Sun, H. Zhang, C. Yuan, Y. Wei et al., Preparation strategies and applications of MXene-polymer composites: a review. Macromol. Rapid Commun. 42, e2100324 (2021). 10.1002/marc.202100324 - PubMed
-
- S. Seyedin, S. Uzun, A. Levitt, B. Anasori, G. Dion et al., MXene composite and coaxial fibers with high stretchability and conductivity for wearable strain sensing textiles. Adv. Funct. Mater. 30, 1910504 (2020). 10.1002/adfm.201910504
-
- X. Qu, S. Wang, Y. Zhao, H. Huang, Q. Wang et al., Skin-inspired highly stretchable, tough and adhesive hydrogels for tissue-attached sensor. Chem. Eng. J. 425, 131523 (2021). 10.1016/j.cej.2021.131523
-
- R. Liu, J. Li, M. Li, Q. Zhang, G. Shi et al., MXene-coated air-permeable pressure-sensing fabric for smart wear. ACS Appl. Mater. Interfaces 12, 46446–46454 (2020). 10.1021/acsami.0c11715 - PubMed
-
- X. Sun, K. Shao, T. Wang, Detection of volatile organic compounds (VOCs) from exhaled breath as noninvasive methods for cancer diagnosis. Anal. Bioanal. Chem. 408, 2759–2780 (2016). 10.1007/s00216-015-9200-6 - PubMed
Publication types
LinkOut - more resources
Full Text Sources