Single-molecule force spectroscopy: optical tweezers, magnetic tweezers and atomic force microscopy
- PMID: 18511917
- PMCID: PMC3397402
- DOI: 10.1038/nmeth.1218
Single-molecule force spectroscopy: optical tweezers, magnetic tweezers and atomic force microscopy
Abstract
Single-molecule force spectroscopy has emerged as a powerful tool to investigate the forces and motions associated with biological molecules and enzymatic activity. The most common force spectroscopy techniques are optical tweezers, magnetic tweezers and atomic force microscopy. Here we describe these techniques and illustrate them with examples highlighting current capabilities and limitations.
Figures
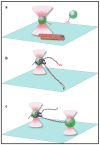
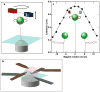
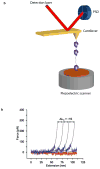
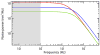
Similar articles
-
Single-molecule force spectroscopy using the NanoTracker optical tweezers platform: from design to application.Curr Pharm Biotechnol. 2009 Aug;10(5):467-73. doi: 10.2174/138920109788922164. Curr Pharm Biotechnol. 2009. PMID: 19689314
-
An Introduction to Magnetic Tweezers.Methods Mol Biol. 2024;2694:375-401. doi: 10.1007/978-1-0716-3377-9_18. Methods Mol Biol. 2024. PMID: 37824014
-
High-resolution, single-molecule measurements of biomolecular motion.Annu Rev Biophys Biomol Struct. 2007;36:171-90. doi: 10.1146/annurev.biophys.36.101106.101451. Annu Rev Biophys Biomol Struct. 2007. PMID: 17328679 Free PMC article. Review.
-
Subpiconewton dynamic force spectroscopy using magnetic tweezers.Biophys J. 2008 Mar 15;94(6):2343-8. doi: 10.1529/biophysj.107.121673. Epub 2007 Dec 7. Biophys J. 2008. PMID: 18065448 Free PMC article.
-
Interrogating biology with force: single molecule high-resolution measurements with optical tweezers.Biophys J. 2013 Sep 17;105(6):1293-303. doi: 10.1016/j.bpj.2013.08.007. Biophys J. 2013. PMID: 24047980 Free PMC article. Review.
Cited by
-
DNA Origami Seesaws as Comparative Binding Assay.Chembiochem. 2016 Jun 16;17(12):1093-6. doi: 10.1002/cbic.201600059. Epub 2016 May 6. Chembiochem. 2016. PMID: 27038073 Free PMC article.
-
Nanomechanics of G-quadruplexes within the promoter of the KIT oncogene.Nucleic Acids Res. 2021 May 7;49(8):4564-4573. doi: 10.1093/nar/gkab079. Nucleic Acids Res. 2021. PMID: 33849064 Free PMC article.
-
Mechanotransduction: use the force(s).BMC Biol. 2015 Jul 4;13:47. doi: 10.1186/s12915-015-0150-4. BMC Biol. 2015. PMID: 26141078 Free PMC article.
-
Emerging Diamond Quantum Sensing in Bio-Membranes.Membranes (Basel). 2022 Sep 30;12(10):957. doi: 10.3390/membranes12100957. Membranes (Basel). 2022. PMID: 36295716 Free PMC article. Review.
-
Force-FAK signaling coupling at individual focal adhesions coordinates mechanosensing and microtissue repair.Nat Commun. 2021 Apr 21;12(1):2359. doi: 10.1038/s41467-021-22602-5. Nat Commun. 2021. PMID: 33883558 Free PMC article.
References
-
- Cluzel P, et al. DNA: An extensible molecule. Science. 1996;271:792–794. - PubMed
-
- Kishino A, Yanagida T. Force measurements by micromanipulation of a single actin filament by glass needles. Nature. 1988;334:74–76. - PubMed
-
- Smith SB, Finzi L, Bustamante C. Direct mechanical measurements of the elasticity of single DNA molecules by using magnetic beads. Science. 1992;258:1122–6. - PubMed
-
- Kim SJ, Blainey PC, Schroeder CM, Xie XS. Multiplexed single-molecule assay for enzymatic activity on flow-stretched DNA. Nat Methods. 2007;4:397–399. - PubMed
Publication types
MeSH terms
Substances
Grants and funding
LinkOut - more resources
Full Text Sources
Other Literature Sources