On this page you can learn more about:
- Inner Loop Control
- Auto Flight System
- Trajectory Generation
- Trajectory Control
- Automatic Take-Off and Landing (ATOL)
- System Automation
Inner Loop Control
Key Facts:
- Linear Baseline Controller
- Certifiable control system with guaranteed stability, robustness and performance characteristics
- High modularity with interface for incorporation of adaption laws or sensor-based nonlinear control methodologies and possibility to adapt to different aircraft configurations
- Envelope protections for aerodynamic and structural integrity
- Consideration of real system effects during gain design
The inner loop controller constitutes one of the most critical parts of the automatic control chain. The inner loop controller has to cope with the most challenging requirements on controller performance and dynamics as well as with the highest requirements on reliability and robustness against model uncertainties and disturbances as
- Time Delays introduced by avionic system
- Sensor Displacement (e.g. distortion of acceleration measurements)
- Model Uncertainties
- A/C Structural excitation
- Actuator dynamics (rate limitations, dead zone effects etc.)
- Dynamic Atmosphere
- Weight and balance (center of gravity position influence)
At the Institute of Flight System Dynamics, research is conducted towards an inner loop controller that is applicable to multiple standard aircraft configurations such as fixed wing CS-23, General Aviation, as well as Fixed Wing light UAV.
Since the inner loop controller relies highly on the aircraft dynamics and configuration setting emphasis is given to the development of a generic controller structure and highly automated gain design process that allows an easy adaption to different aircraft configurations.
Generic interfaces facilitate the application of the controller structure in multiple aircraft configurations. A high degree of automation in the Gain Design Process allows to easily adjust the configuration-specific (aircraft dynamics) and application-specific (performance requirements) controller parameters (e.g. gain sets) as well as the adjustment of the envelope protections for aerodynamic and structural integrity.
To the requirements driven development, the consideration of the above mentioned real system effects, which highly influence inner loop control performance and robustness, is incorporated into the structural as well the gain design process. The controller structure provides an interface for incorporation of adaption laws or sensor-based nonlinear control methodologies to account for uncertainties or fault scenarios as structural changes caused by damages.
Auto Flight System
Key Facts:
- Research and development driven by increased importance and availability of automatic flight control for manned and unmanned aircraft
- A single modular and configurable system providing necessary functionalities with guaranteed stability, performance and robustness characteristics for a large range of automatic flight control applications, fixed-wing aircraft configurations and sensor environments
- Utilization of the advantages of model-based system and software development and of strategic partnerships to reach and go beyond the state-of-the-art of CS-23 digital flight control
Increased Digitalization of CS-23 and UAV Avionics
In the last decades, an increased digitalization of the avionics in commuter, business and general aviation aircraft has taken place. Automatic flight control functions and navigation aids previously only available in commercial airliners can now be found in many general aviation autopilots. This development is mainly due the availability of a number of new affordable, reliable and high-quality components, e.g. MEMS (micro-electro-mechanical system) accelerometers and gyros, performant and robust processor for embedded systems, as well as compact but intelligent and high-performant electro-mechanical actuators. These affordable technologies have also led to the large increase in development and operation of remotely controlled or automated smaller unmanned aircraft. Further, tools for the development of software for safety-critical applications has become more affordable and integrated with development process requirements, a change driven by increased demand from industries outside the aerospace sector (e.g. automotive).
These evolutions have made new approaches to the research and development of affordable and product relevant digital flight control applications, under the cost and system engineering specific constraints of the CS-23 sector, possible. Together with strategic small and medium-sized partner companies, specialized in embedded systems, sensor and actuation technologies, the Institute of Flight System Dynamics aims at strengthening the cooperative systems engineering effort and the research and development of product relevant flight control applications.
Functionalities and Design Challenges
Automatic flight control functions provided include basic attitude hold modes, flight path control (vertical speed/flight path angle and/or heading/track angle), altitude capture and hold, speed by pitch and automatic thrust control, VOR navigation as well as standard ILS approach capture and track. For each function, the operational conditions are to be monitored. To what extent is continued operation in degraded sensor environments possible (temporary or total loss of signals)? In presence of a control objective conflict, e.g. a combination of airspeed and flight path that cannot be achieved due to saturated thrust, how can an active prioritization of objectives ensure a deterministic behavior in all situations? How may protections and limitations of critical flight parameters such as airspeed and load factors ensure the aerodynamic and structural integrity of the aircraft, without sacrificing system performance? How is the system best implemented in order to ensure testability? How may functional elements be capsulated and verified for correct functionality, robustness and performance?
Challenges and Opportunities of Model-Based Development
Designing and building a complex, digital flight control system is a great challenge for a small and distributed team. The use of a model-based approach for the application development offers great opportunities for rapid prototyping, early validation, automation of verification activities and testing and managing interfaces. How do we create synergies between the early desktop simulation and later verification activities in the hardware-in-the-loop simulation and flight testing, by defining and re-using test cases and procedures? How do we model and formalize requirements and how may we use those models for an online monitoring of the system? These questions and many more are part of the research and development activities related to the application driven automatic flight control at the Institute of Flight System Dynamics.
Trajectory Generation
Key Facts
- Generating trajectory parameters and relative kinematics
- Achievability of trajectories
- Deterministic trajectory behavior
- Dynamic flightplan editing
- Voice command inputs
The research field of trajectory generation deals with the online calculation of a flight path parameterization. This includes the trajectory parameters itself and the relative kinematics between aircraft and trajectory. The objective is a parameterization down to the second order. There exist different approaches to achieve this task, but the research focus in this field lies on application driven systems. Therefore, the trajectory generation has to fulfill additional, sometimes conflicting, requirements:
- The trajectory has to be achievable by the aircraft in all occurring situations. Therefore, the trajectory planning algorithm has to consider aircraft performance limitations (maximum climb rate, maximum speed, maximum altitude, etc…), environment conditions (e.g. wind speed, turbulences) and has to react adequately on changes of those parameters.
- The system has to act completely deterministic respecting the trajectory generation, since the operator and other air traffic participants need to know exactly, what the aircraft will do.
- The trajectory generation algorithm has to guarantee a solution for the needed parameters in a predefined sample time. The sample time is driven by the trajectory controller, which needs the actual relative kinematics and trajectory data each time step.
- The trajectory generation has to react dynamically in flight on flight plan changes. Due to the application driven approach, the integration into civil airspace requires an adequate reaction on ATC commands or on current air traffic. To fulfill this task, one research field is the automatic control of an UAV via voice commands, so that a direct reaction on ATC commands is possible.

Trajectory Control
Key Facts:
- Generic interface for multiple applications in manned and unmanned flight
- Nonlinear error dynamics for improved performance
- Development under consideration of certification and safety aspects
In the recent years, the degree of automation for path and trajectory flight has been steadily increased. As a consequence, the need to follow these pre-specified paths and trajectories has emerged rapidly for manned applications as well as for unmanned aerial vehicles. At the Institute of Flight System Dynamics, research is conducted towards trajectory / path following controllers, which are developed under consideration of existing aerospace standards and take into account modern nonlinear control methods.
At the institute, various different applications require such controllers. Hence, a generic interface containing trajectory information and the displacements of the airborne system with respect to the trajectory. In the figure, these displacements and its derivatives in the trajectory frame are defined.

Employing such a generic interface enables the controller to be utilized for different functionalities ranging from actual trajectory / path following in waypoint-based flight to specific landing routes. Hence, all functionalities, which are able to provide data for this generic interface, can utilize this controller.
Since various applications also imply diverse aircraft dynamics, the command structure to the inner loop controller is also designed in a generic way, such that the controller can be used for different configurations.
The research on trajectory control is based on nonlinear error dynamics between desired trajectory and airborne system. Different control methodologies are investigated in order to take into account modern control concepts such as nonlinear dynamic inversion as well as certification requirements, which are usually derived from “traditional” flight control approaches.
The infrastructure of the institute enables extensive testing incorporating hardware-in-the-loop simulations with safety critical hardware and hence, the prerequisites for requirements-based control design and model-based software development are available. Evaluation of sensor availability and alternate procedures in case of faults are also part of the research on trajectory control and emphasize the institute’s demand to address safety-critical aspects of control design.
Automatic Take-Off and Landing (ATOL)
Key Facts:
- Automatic Take-Off and Landing of manned and unmanned light aircraft
- Consideration of disturbances, technical errors and robustness in design and operation
- Stochastic investigation of system performance and model based requirement engineering
- Safety- and certification-related development
Take-off and, especially, landing maneuvers are among the most challenging procedures in flight operation of fixed wing aircraft. Due to close proximity to the ground, failures or disturbances may easily lead to abnormal ground contact and thus fatal accidents. It is not surprising that many accidents occur during these flight phases. One of the Institute’s objective is therefore to improve the capabilities of aircraft to perform take-off and landing maneuvers automatically. Automatic landing maneuvers are treated from intermediate approach down to roll-out and full-stop on the runway. Both manned and unmanned systems are subject of research, either to provide a supporting safety system or to increase the level of autonomy, respectively.
In contrast to quite common automatic landing systems for commercial airliners, additional challenges arise with respect to light aircraft due to a higher sensitivity to atmospheric disturbances. This leads to more demanding requirements for robustness of the flight guidance and automatic control system. Stochastic model based investigation methods are thereby used to derive physically motivated performance requirements.
In order to increase the availability of the ATOL-System even in the presence of failures or disturbances, the total system error is monitored and predicted rather than assessing the flight technical error and navigation error separately. This leads to less conservative abort criteria without compromising the level of safety.
Finally, by using alternative positioning systems, further benefits arise from a reduction of costs and an increased availability on small- and medium-sized airfields. For instance, take-off and landing maneuvers can be performed on the basis of differential GPS or by means of an onboard camera instead of making use of the widely established but expensive landing aid ILS.
Striving for compliance with the demanding requirements imposed by aviation authorities and paving the way for later certification of the results, the Institute’s infrastructure is used to establish requirement based development, safety assessment, and extensive testing of soft- and hardware on ground and in flight.
The rising expertise in the field of automatic take-off and landing for fixed wing aircraft on runways is moreover used to design and investigate landing scenarios for extraterrestrial landers. High availability and reliability are essential for this kind of operations.
System Automation
Key Facts:
- Generic Interface for configuration independent automation
- Switching between different operational modes
- System monitoring
- Secondary Flight System Handling
In modern unmanned aerial systems, there are many controllers which are responsible for different parts of a flight mission or for controlling the aircraft on different levels. At the institute many applications use the same controller structure. Therefore a generic interface containing inputs and outputs is developed for the part of the system automation, which is independent of the configuration. A simplified example structure is shown in the picture below.
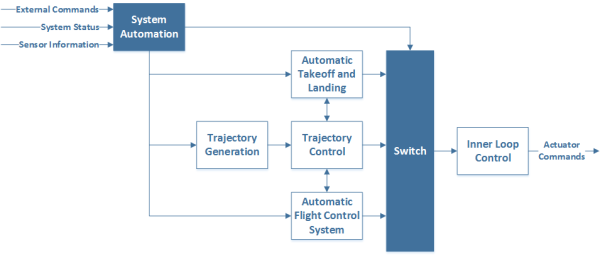
To perform a given flight mission the flight controllers (e.g. Trajectory Generation, Trajectory Control, Automatic Flight Control System or Automatic Takeoff and Landing) have to be activated and connected in a certain way. To ensure the correct switching a superior system automation logic is needed. The switching between different control modes can for example be based on external commands from a flight operator, system status information like the availability of the data link or sensor information e.g. the quality of the position from the navigation system. This information is merged within the system automation and then commands for the controllers and switches are generated.
The system monitoring within the system automation generates information which are depended on the sensor or signal values. One part are the range checks which are executed to determine if a signal (mostly sensor signals) are outside of their expected range even though the integrity information indicates that they are reliable. To compensate sensor or system failures which are detected from the system monitoring a degradation concept is developed. For example if the data link is lost for a certain time, the aircraft can automatically but in a deterministic behavior return to the home base.
In an unmanned aerial system also secondary flight systems, e.g. the flaps or gear, are automated. They have a direct influence on the aircraft’s envelope, which can be based on aerodynamic or structural limits. To always operate the aircraft in the specified envelope, certain limits within the flight controllers have to be adjusted in accordance the status or position of the secondary flight controls.
To handle the complexity during the development of these algorithms a model-based software development process is established at the institute. For the system automation finite-state machines are used. To cope with growing complexity they are divided into small functions. Those small state machines are than for example tested using formal methods to identify hard to find design errors.
Team
Zoe Mbikayi, Agnes Steinert, Alexander ZollitschPublications
Development of a Navigation Solution for an Image Aided Automatic Landing System", in ION PNT, 2013. | , "|
Automatic Landing System using SBAS and Electro Optical Sensor Measurement Data", in UAV World Conference, 2013. | , "|
Autoland Controller Design Procedure based on Total System Error Concept for a Small UAV Aircraft", in AIAA Guidance, Navigation, and Control (GNC) Conference, 2013. | , "