A variety of innovative external and internal patches that employ flexible and stretchable materials, machine learning, and other tactics to monitor heart activity and function may eventually lessen the impact of disease
Cardiovascular disease may be the world’s leading killer of men and women, but new technologies are in development that could help lessen its impact. Among them are a variety of innovative external and internal patches that employ flexible and stretchable materials, machine learning, and other tactics to monitor heart activity and function, and in some cases to provide on-the-spot treatment.
Examples of these technologies include wearable external patches to perform continuous ultrasound imaging of the heart, even as a patient exercises; a chest-worn patch that collects electrocardiogram (ECG) and seismocardiogram (SCG) data over multiple weeks and transmits the data via app to the patient’s health care team; and a temporary on-the-heart patch to track heart function following a cardiac event and deliver therapeutic electrical stimulations as needed.
Ultrasound patch
A typical cardiac ultrasound, also known as transthoracic echocardiography (TTE), currently requires a trained technologist to run a bulky probe over a lying patient’s chest to visualize and evaluate heart function. By comparison, a wearable cardiac ultrasound imager (Figure 1) under development at the University of California San Diego (UCSD) needs neither this probe nor a probe operator. Instead, it is a small, soft, flexible patch the size of a postage stamp that sticks onto the chest of a patient, who can then move about as the patch collects TTE data, according to project leaders Ray Wu and Hao Huang (Figure 2) of the UCSD Department of Nanoengineering. Wu and Huang are two of the coauthors of a Nature article describing the patch [1].
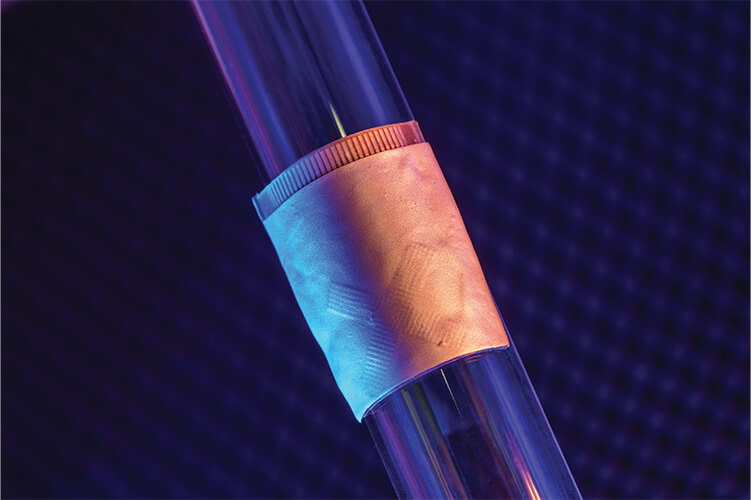
Figure 1. UCSD’s wearable ultrasound device, about the size of a postage stamp, attaches to the chest and provides a continuous recording of heart structure and function during normal activity, including during exercise, for up to 24 hours. (Photo courtesy of Sheng Xu Laboratory at UCSD.)
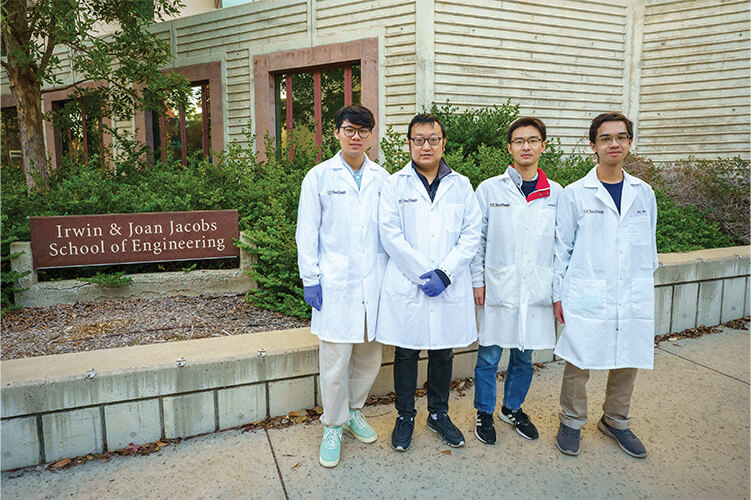
Figure 2. UCSD study coauthors (left to right) Hao Huang, Hongjie Hu, Xiaoxiang Gao, and Ray Wu, who are all members of the lab of Sheng Xu, Ph.D., associate professor of nanoengineering at the UCSD. (Photo courtesy of David Baillot/UCSD Jacobs School of Engineering.)
“Overall, our patch just makes everything more convenient and also adds some new capabilities that you normally cannot do with a handheld probe,” Wu said. The device incorporates a ground electrode layer on the bottom and electromagnetic-noise-shielding layers on top, with four layers of liquid metal electrodes and a transducer layer sandwiched between. “The liquid metal can conduct, and four layers allow us to have a high density of connections. The transducer layer is an orthogonal array of ultrasound transducer elements that we have diced apart and connected to the liquid metal electrodes, so even though these individual transducer elements are locally rigid, they are connected by the liquid metal so the whole device is stretchable,” Wu explained (Figure 3).
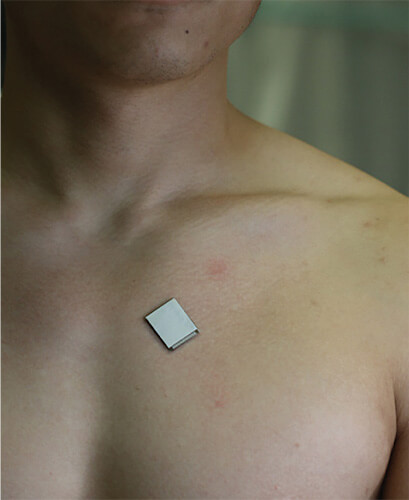
Figure 3. The UCSD device, shown on the body, is flexible and functional, thanks in part to an orthogonal array of ultrasound transducer elements connected to liquid metal electrodes. (Photo courtesy of Sheng Xu Laboratory at UCSD.)
Each arm of the orthogonal transducer array acts independently, so rather than the operation of a traditional ultrasound that requires a technician to rotate the ultrasound probe 90° to get different cross-sectional views of the heart, such as parasternal long axis and parasternal short axis views, the UCSD device is able to image both views from the same position simultaneously. Because the flexible device sticks and conforms to the body, it can also perform ultrasound imaging while the patient exercises, something that is not possible with traditional ultrasound. Huang added, “This is accomplished because the device not only moves with the body to eliminate relative motion that might induce motion artifacts, but also incorporates a 3D scanning camera to determine the curvature of the chest surface and know the exact positions of the transducer elements in the device.”
A 24-hour test on a subject demonstrated that it has the potential to do long-term monitoring as well, Wu reported. To deal with the large amount of data the device would collect over such a long span, the researchers integrated artificial-intelligence and deep-learning capabilities that can process the data automatically and generate long-term quantitative monitoring of the heart’s performance, such as cardiac output, and ejection fraction (percentage of the volume being ejected per stroke), he said.
The researchers are now refining the device’s ultrasound images so they more closely approach the clarity of those produced by traditional ultrasound machines and are planning to convert data transmission from the current wired connection to a wireless one, Huang said. Concurrently, the spinoff company Softsonics LLC of San Diego, CA, USA, co-founded by senior study author and UCSD associate professor of nanoengineering Sheng Xu, Ph.D., plans to commercialize the technology. (Softsonics won a National Institutes of Health (NIH) National Technology Accelerator Challenge prize in 2022 for a wearable ultrasound/electrochemical sensor for maternal health surveillance [2].)
This technology could have uses beyond heart monitoring, Wu suggested. “Actually, some doctors have already asked us if we can image multiple sites at once to perhaps find correlations between different organs. So yes, I think this device can be used in different ways and target any organ.”
ECG and SCG monitoring
A research group at the University of Missouri is creating a wearable patch that uses circuitry and electrodes to track ECG and SCG data as a way to help clinicians determine a patient’s risk for heart disease. “Heart disease is the leading cause of death in the USA, but a high percentage of heart disease—on the order of 90%—is treatable if we can detect it at an early stage. This is a major reason why we want to develop a wearable heart monitor that provides long-term and continuous heart monitoring,” said lead researcher Zheng Yan, Ph.D., associate professor of chemical and BME, and mechanical and aerospace engineering (Figure 4). With the help of a $2.6 million NIH grant, Yan and his group are developing “a wearable heart monitor that can work for a long time: more than two weeks, perhaps as long as one month,” Yan said.
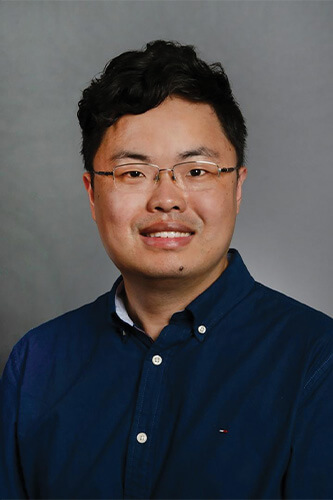
Figure 4. Zheng Yan, Ph.D., associate professor of chemical and BME, and mechanical and aerospace engineering. (Photo courtesy of University of Missouri.)
For this long-term, skin-adhered device, materials development was a primary concern. “The existing wearable heart monitors typically are made of nonporous materials, but we are human beings and we sweat a lot, and with nonporous materials, water can easily accumulate between the wearable patch and the human skin and can cause trouble, such as itching and inflammation,” Yan explained. “With our background in materials science [3], [4], we are developing a new structure with what we call phase separation, and that includes interconnected pores so that sweat can easily evaporate through the pores.” They are also introducing antibacterial and antiviral capabilities into the material, so they are safer for long-term wear, and are useful for avoiding bacterial accumulation that could potentially affect data acquisition, he noted.
The phase-separation approach also allows the patch to overcome mechanical-electrical issues that might degrade or affect collected electrical signals for accurate heart monitoring. “Our new materials are insensitive mechanical deformations, such as mechanical-electrical decoupling, so we can stretch, bend, and twist the patch, and the electrical performance remains very stable,” Yan described. His research group is collaborating with experts in electrical engineering and computer science to develop a machine-learning algorithm to further reduce any remaining motion artifacts. “We are planning to publish the details of the algorithm and material within the next year,” he said.
Yan and his group have already developed all of the device components, including an adhesive that is suitable for long-term wear, but is also easily removable (Figure 5). “Our smart adhesive attaches the bottom of the device to the chest skin at body temperature, but loses its adhesion at low temperatures, so it can very easily peel off,” he said. They are now building a prototype of the entire wearable system, and in about a year hope to begin testing it on patients through a collaboration with a hospital physician. “Our goal is to address a real problem in the clinic,” he added.
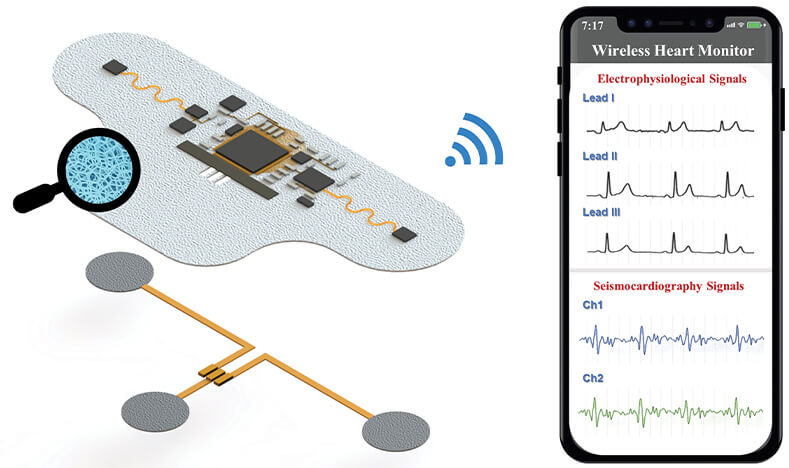
Figure 5. Yan’s group is developing a wearable chest patch that can collect ECG and SCG data over multiple weeks, and transmit the data via app to the patient’s health care team. The patch incorporates interconnected pores to allow sweat dissipation, as well as antibacterial and antiviral capabilities. (Image courtesy of the Yan Group.)
Postevent patch
At George Washington University in Washington, DC, USA, and Northwestern University in Evanston, IL, USA, researchers are designing an implantable patch to monitor patients who have already undergone treatment for a cardiac episode. “Heart disease is the leading cause of death in the United States, and one-third of the deaths are actually due to complications in the first weeks following a traumatic heart-related event. That is what drives us to develop better platforms that can improve the patient’s survival,” said Zhiyuan Chen, Ph.D., (Figure 6) lead author of an article in Science Advances that describes the work [5]. During this research, Chen was a doctoral student in the lab of Luyao Lu, Ph.D., at George Washington University. Lu co-led the study with biomedical engineer and experimental cardiologist Igor Efimov, Ph.D., of Northwestern University. (This work was also inspired by Efimov’s previous work with John A. Rogers, Ph.D., of Northwestern’s McCormick School of Engineering and Applied Science.)
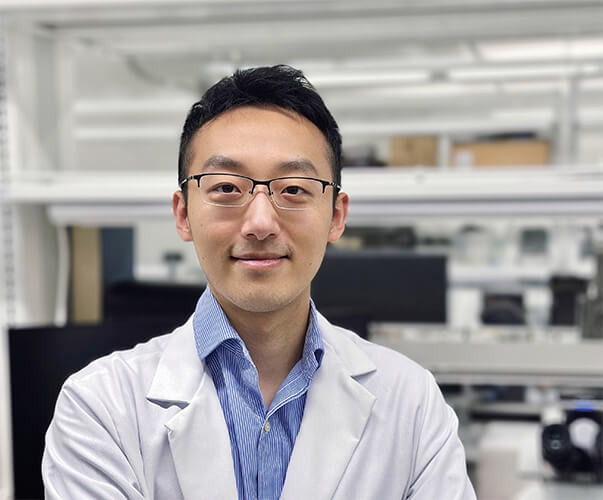
Figure 6. Zhiyuan Chen, Ph.D., who is the lead author of an article describing a dissolvable cardiac device for use after a cardiac event, such as a heart attack. Chen conducted the work as a doctoral student in the George Washington University lab of biomedical engineer Luyao Lu, Ph.D., who co-led the study with biomedical engineer and experimental cardiologist Igor Efimov, Ph.D., of Northwestern University. Chen is now starting a postdoctoral position at Stanford University in California. (Photo courtesy of Ji Qi.)
As described in Science Advances, the research group developed a dissolvable cardiac device (Figure 7) that is placed directly on the heart to temporarily perform monitoring services and, when needed, deliver electrical stimulation to regulate the heart rhythm or reset the heartbeat, Chen said. After a set amount of time, which may be several weeks, the device dissolves away in the body. As of November 2023, the researchers had a patch that continued working for about a week after attachment, and they currently are working to extend that timeframe.
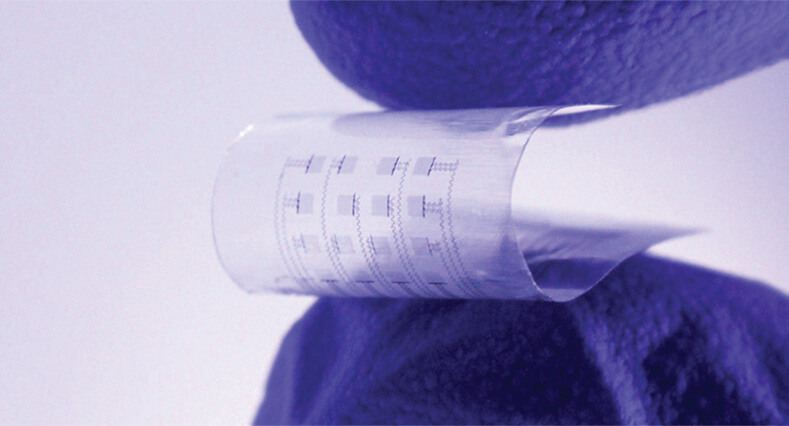
Figure 7. This device, which is flexible and equipped with sensors and actuators, is designed for the critical period following a cardiac surgery or catheter-based therapy. It sits directly on the heart, where it temporarily performs monitoring services and, when needed, delivers electrical stimulation to regulate the heart rhythm or reset the heartbeat. (Photo courtesy of Northwestern University/George Washington University.)
The device is built on FDA-approved biocompatible and biodegradable materials, including molybdenum, a naturally occurring element in the human body; and PLGA, or poly(lactic-glycolic acid), which is a widely used polymer in drug-delivery technology. However, that presented some challenges, Chen said. “One is that such materials are sensitive to moisture, heat, chemicals, and other environmental factors, so it is more challenging to process them, especially when patterning them into small feature sizes such as nanoscale.” That led the researchers to develop a new fabrication strategy that combines photolithography, electron beam lithography, and soft lithography. “Briefly, we use an intermediate material that is chemically different from other materials, but can withstand all processing under harsh conditions, and finally release the device at a controllable rate through chemical selectivity,” he explained.
The soft device is equipped with an array of sensors and actuators that can image the electrical activity of the heart to show which areas are functioning well and which areas are not, then deliver electrical stimulation to that site when needed. “It can do both at the same time, so it can be a continuous process of monitoring and treatment, and the treatment effects can also be evaluated in real time,” Chen said. In addition, the device is transparent, which allows doctors to have a clear view of the heart when necessary during procedures.
So far, the research group has tested the thin, transparent, postage-stamp-sized device on rats and on human hearts (not on human patients). Currently, data transmission from the device is wired, Chen noted. “We still use flexible ACF (anisotropic conductive film) cable to power and control the device, but in the future, we plan to make it totally wireless.”
Chen sees many possibilities ahead. For one, this approach can achieve nanoscale transient patterns and do so at a high yield, which opens the door to the large-scale integration of other miniaturized biomedical devices. “Dr. Lu already envisions that this can be applied to brain diseases, such as Parkinson’s and Alzheimer’s, perhaps to image and treat them,” Chen said. “And I envision potentially adding drug-delivery functionality to this dissolvable device: Based on the feedback of the organ or concentration changes of biomarkers, it could deliver a certain amount of the drug in a timely manner for precision medicine. Or because this device is transparent, we could perhaps integrate biodegradable LEDs on top to achieve the optogenetic studies in vivo, and later they would just disappear. That would be amazing.”
He added, “It will be very exciting to integrate more components and extend the functions of this platform.”
References
- H. Hu et al., “A wearable cardiac ultrasound imager,” Nature, vol. 613, no. 7945, pp. 667–675, Jan. 2023, doi: 10.1038/s41586-022-05498-z.
- National Institute of Biomedical Imaging and Bioengineering. (Jul. 28, 2022). NIH Announces Prize Winners of Maternal Health Diagnostics Challenge. Accessed: Nov. 28, 2023. [Online]. Available: https://www.nibib.nih.gov/news-events/newsroom/nih-announces-prize-winners-maternal-health-diagnostics-challenge
- G. Zhao et al., “Laser-scribed conductive, photoactive transition metal oxide on soft elastomers for Janus on-skin electronics and soft actuators,” Sci. Adv., vol. 8, no. 25, p. eabp9734, Jun. 2022, doi: 10.1126/sciadv.abp9734.
- B. Sun et al., “Gas-permeable, multifunctional on-skin electronics based on laser-induced porous graphene and sugar-templated elastomer sponges,” Adv. Mater., vol. 30, no. 40, p. e1804327, Dec. 2018, doi: 10.1002/adma.201804327.
- Z. Chen et al., “Soft, bioresorbable, transparent microelectrode arrays for multimodal spatiotemporal mapping and modulation of cardiac physiology,” Sci. Adv., vol. 9, no. 27, p. eadi0757, Jul. 2023, doi: 10.1126/sciadv.adi0757.