Short-term synaptic plasticity in emerging devices for neuromorphic computing
- PMID: 36950108
- PMCID: PMC10025973
- DOI: 10.1016/j.isci.2023.106315
Short-term synaptic plasticity in emerging devices for neuromorphic computing
Abstract
Neuromorphic computing is a promising computing paradigm toward building next-generation artificial intelligence machines, in which diverse types of synaptic plasticity play an active role in information processing. Compared to long-term plasticity (LTP) forming the foundation of learning and memory, short-term plasticity (STP) is essential for critical computational functions. So far, the practical applications of LTP have been widely investigated, whereas the implementation of STP in hardware is still elusive. Here, we review the development of STP by bridging the physics in emerging devices and biological behaviors. We explore the computational functions of various STP in biology and review their recent progress. Finally, we discuss the main challenges of introducing STP into synaptic devices and offer the potential approaches to utilize STP to enrich systems' capabilities. This review is expected to provide prospective ideas for implementing STP in emerging devices and may promote the construction of high-level neuromorphic machines.
Keywords: Applied computing; Devices; Engineering.
© 2023 The Author(s).
Conflict of interest statement
The authors declare no competing interests.
Figures
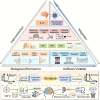
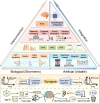
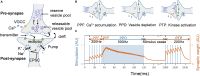
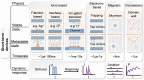
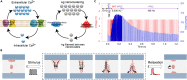
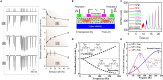
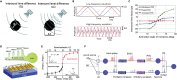
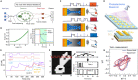
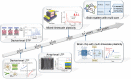
Similar articles
-
Self-Doping Memristors with Equivalently Synaptic Ion Dynamics for Neuromorphic Computing.ACS Appl Mater Interfaces. 2019 Jul 10;11(27):24230-24240. doi: 10.1021/acsami.9b04901. Epub 2019 Jun 6. ACS Appl Mater Interfaces. 2019. PMID: 31119929
-
Truly Concomitant and Independently Expressed Short- and Long-Term Plasticity in a Bi2 O2 Se-Based Three-Terminal Memristor.Adv Mater. 2019 Jan;31(3):e1805769. doi: 10.1002/adma.201805769. Epub 2018 Nov 20. Adv Mater. 2019. PMID: 30461090
-
Solution-Processed Polymer Memcapacitors with Stimulus-Controlled and Evolvable Synaptic Functionalities: From Short-Term Plasticity to Long-Term Plasticity to Metaplasticity.ACS Appl Mater Interfaces. 2024 Sep 11;16(36):47996-48004. doi: 10.1021/acsami.4c09593. Epub 2024 Sep 2. ACS Appl Mater Interfaces. 2024. PMID: 39221579
-
Emerging memristive neurons for neuromorphic computing and sensing.Sci Technol Adv Mater. 2023 Apr 19;24(1):2188878. doi: 10.1080/14686996.2023.2188878. eCollection 2023. Sci Technol Adv Mater. 2023. PMID: 37090846 Free PMC article. Review.
-
Biological function simulation in neuromorphic devices: from synapse and neuron to behavior.Sci Technol Adv Mater. 2023 Mar 10;24(1):2183712. doi: 10.1080/14686996.2023.2183712. eCollection 2023. Sci Technol Adv Mater. 2023. PMID: 36926202 Free PMC article. Review.
Cited by
-
TiN/Ti/HfO2/TiN memristive devices for neuromorphic computing: from synaptic plasticity to stochastic resonance.Front Neurosci. 2023 Sep 19;17:1271956. doi: 10.3389/fnins.2023.1271956. eCollection 2023. Front Neurosci. 2023. PMID: 37795180 Free PMC article.
-
Volatile tin oxide memristor for neuromorphic computing.iScience. 2024 Jul 9;27(8):110479. doi: 10.1016/j.isci.2024.110479. eCollection 2024 Aug 16. iScience. 2024. PMID: 39129832 Free PMC article.
-
An overview of critical applications of resistive random access memory.Nanoscale Adv. 2024 Sep 9;6(20):4980-5006. doi: 10.1039/d4na00158c. Online ahead of print. Nanoscale Adv. 2024. PMID: 39263252 Free PMC article. Review.
-
Distinctive properties of biological neural networks and recent advances in bottom-up approaches toward a better biologically plausible neural network.Front Comput Neurosci. 2023 Jun 28;17:1092185. doi: 10.3389/fncom.2023.1092185. eCollection 2023. Front Comput Neurosci. 2023. PMID: 37449083 Free PMC article. Review.
-
Low power nanoscale S-FED based single ended sense amplifier applied in integrate and fire neuron circuit.Sci Rep. 2024 May 9;14(1):10691. doi: 10.1038/s41598-024-61224-x. Sci Rep. 2024. PMID: 38724680 Free PMC article.
References
Publication types
LinkOut - more resources
Full Text Sources