Ablation of STAT3 in Purkinje cells reorganizes cerebellar synaptic plasticity in long-term fear memory network
- PMID: 33459594
- PMCID: PMC7813544
- DOI: 10.7554/eLife.63291
Ablation of STAT3 in Purkinje cells reorganizes cerebellar synaptic plasticity in long-term fear memory network
Abstract
Emotional memory processing engages a large neuronal network of brain regions including the cerebellum. However, the molecular and cellular mechanisms of the cerebellar cortex modulating the fear memory network are unclear. Here, we illustrate that synaptic signaling in cerebellar Purkinje cells (PCs) via STAT3 regulates long-term fear memory. Transcriptome analyses revealed that PC-specific STAT3 knockout (STAT3PKO) results in transcriptional changes that lead to an increase in the expression of glutamate receptors. The amplitude of AMPA receptor-mediated excitatory postsynaptic currents at parallel fiber (PF) to PC synapses was larger in STAT3PKO mice than in wild-type (WT) littermates. Fear conditioning induced long-term depression of PF-PC synapses in STAT3PKO mice while the same manipulation induced long-term potentiation in WT littermates. STAT3PKO mice showed an aberrantly enhanced long-term fear memory. Neuronal activity in fear-related regions increased in fear-conditioned STAT3PKO mice. Our data suggest that STAT3-dependent molecular regulation in PCs is indispensable for proper expression of fear memory.
Keywords: AMPA receptors; Purkinje cells; STAT3; fear memory network; mouse; neuroscience.
© 2021, Han et al.
Conflict of interest statement
JH, SK, YK, JC, JK, YL, SY, SK No competing interests declared
Figures
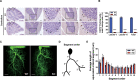
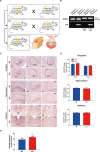
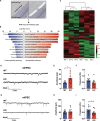
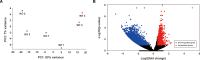
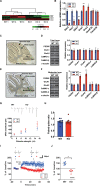
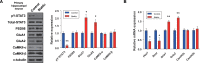
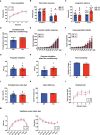
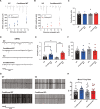
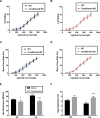
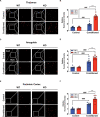
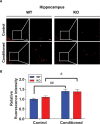
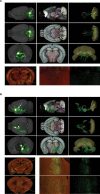
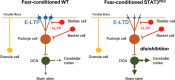
Similar articles
-
Long-Term Depression of Intrinsic Excitability Accompanied by Synaptic Depression in Cerebellar Purkinje Cells.J Neurosci. 2017 Jun 7;37(23):5659-5669. doi: 10.1523/JNEUROSCI.3464-16.2017. Epub 2017 May 11. J Neurosci. 2017. PMID: 28495974 Free PMC article.
-
Properties of 4 Hz stimulation-induced parallel fiber-Purkinje cell presynaptic long-term plasticity in mouse cerebellar cortex in vivo.Eur J Neurosci. 2014 May;39(10):1624-31. doi: 10.1111/ejn.12559. Epub 2014 Mar 26. Eur J Neurosci. 2014. PMID: 24666426
-
Interneuronal NMDA receptors regulate long-term depression and motor learning in the cerebellum.J Physiol. 2019 Feb;597(3):903-920. doi: 10.1113/JP276794. Epub 2018 Nov 24. J Physiol. 2019. PMID: 30382582 Free PMC article.
-
Modulation, Plasticity and Pathophysiology of the Parallel Fiber-Purkinje Cell Synapse.Front Synaptic Neurosci. 2016 Nov 3;8:35. doi: 10.3389/fnsyn.2016.00035. eCollection 2016. Front Synaptic Neurosci. 2016. PMID: 27857688 Free PMC article. Review.
-
Synaptic memories upside down: bidirectional plasticity at cerebellar parallel fiber-Purkinje cell synapses.Neuron. 2006 Oct 19;52(2):227-38. doi: 10.1016/j.neuron.2006.09.032. Neuron. 2006. PMID: 17046686 Review.
Cited by
-
Cerebellar contribution to the regulation of defensive states.Front Syst Neurosci. 2023 Mar 31;17:1160083. doi: 10.3389/fnsys.2023.1160083. eCollection 2023. Front Syst Neurosci. 2023. PMID: 37064160 Free PMC article. Review.
-
Cerebellum and Emotion Memory.Adv Exp Med Biol. 2022;1378:53-73. doi: 10.1007/978-3-030-99550-8_5. Adv Exp Med Biol. 2022. PMID: 35902465
-
Recent Advances in the Understanding of Specific Efferent Pathways Emerging From the Cerebellum.Front Neuroanat. 2021 Dec 16;15:759948. doi: 10.3389/fnana.2021.759948. eCollection 2021. Front Neuroanat. 2021. PMID: 34975418 Free PMC article. Review.
-
VTA-projecting cerebellar neurons mediate stress-dependent depression-like behaviors.Elife. 2022 Feb 14;11:e72981. doi: 10.7554/eLife.72981. Elife. 2022. PMID: 35156922 Free PMC article.
-
Novel Cerebello-Amygdala Connections Provide Missing Link Between Cerebellum and Limbic System.Front Syst Neurosci. 2022 May 13;16:879634. doi: 10.3389/fnsys.2022.879634. eCollection 2022. Front Syst Neurosci. 2022. PMID: 35645738 Free PMC article.
References
-
- Adamaszek M, D'Agata F, Ferrucci R, Habas C, Keulen S, Kirkby KC, Leggio M, Mariën P, Molinari M, Moulton E, Orsi L, Van Overwalle F, Papadelis C, Priori A, Sacchetti B, Schutter DJ, Styliadis C, Verhoeven J. Consensus paper: cerebellum and emotion. The Cerebellum. 2017;16:552–576. doi: 10.1007/s12311-016-0815-8. - DOI - PubMed
Publication types
MeSH terms
Substances
Grants and funding
LinkOut - more resources
Full Text Sources
Other Literature Sources
Molecular Biology Databases
Miscellaneous