Spectral Vegetation Indices to Track Senescence Dynamics in Diverse Wheat Germplasm
- PMID: 32047504
- PMCID: PMC6997566
- DOI: 10.3389/fpls.2019.01749
Spectral Vegetation Indices to Track Senescence Dynamics in Diverse Wheat Germplasm
Abstract
The ability of a genotype to stay green affects the primary target traits grain yield (GY) and grain protein concentration (GPC) in wheat. High throughput methods to assess senescence dynamics in large field trials will allow for (i) indirect selection in early breeding generations, when yield cannot yet be accurately determined and (ii) mapping of the genomic regions controlling the trait. The aim of this study was to develop a robust method to assess senescence based on hyperspectral canopy reflectance. Measurements were taken in three years throughout the grain filling phase on >300 winter wheat varieties in the spectral range from 350 to 2500 nm using a spectroradiometer. We compared the potential of spectral indices (SI) and full-spectrum models to infer visually observed senescence dynamics from repeated reflectance measurements. Parameters describing the dynamics of senescence were used to predict GY and GPC and a feature selection algorithm was used to identify the most predictive features. The three-band plant senescence reflectance index (PSRI) approximated the visually observed senescence dynamics best, whereas full-spectrum models suffered from a strong year-specificity. Feature selection identified visual scorings as most predictive for GY, but also PSRI ranked among the most predictive features while adding additional spectral features had little effect. Visually scored delayed senescence was positively correlated with GY ranging from r = 0.173 in 2018 to r = 0.365 in 2016. It appears that visual scoring remains the gold standard to quantify leaf senescence in moderately large trials. However, using appropriate phenotyping platforms, the proposed index-based parameterization of the canopy reflectance dynamics offers the critical advantage of upscaling to very large breeding trials.
Keywords: canopy reflectance; feature selection; field-based phenotyping; high-throughput phenotyping; hyperspectral remote sensing.
Copyright © 2020 Anderegg, Yu, Aasen, Walter, Liebisch and Hund.
Figures
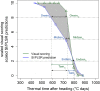
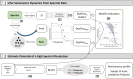
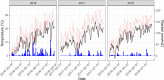

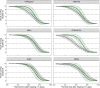
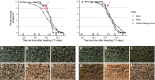
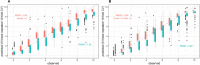
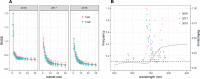
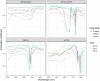
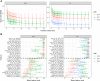
Similar articles
-
Predicting grain protein content of field-grown winter wheat with satellite images and partial least square algorithm.PLoS One. 2020 Mar 11;15(3):e0228500. doi: 10.1371/journal.pone.0228500. eCollection 2020. PLoS One. 2020. PMID: 32160185 Free PMC article.
-
High-Throughput Field Phenotyping Traits of Grain Yield Formation and Nitrogen Use Efficiency: Optimizing the Selection of Vegetation Indices and Growth Stages.Front Plant Sci. 2020 Jan 17;10:1672. doi: 10.3389/fpls.2019.01672. eCollection 2019. Front Plant Sci. 2020. PMID: 32010159 Free PMC article.
-
In-Field Detection and Quantification of Septoria Tritici Blotch in Diverse Wheat Germplasm Using Spectral-Temporal Features.Front Plant Sci. 2019 Oct 25;10:1355. doi: 10.3389/fpls.2019.01355. eCollection 2019. Front Plant Sci. 2019. PMID: 31708956 Free PMC article.
-
Identification of stay-green and early senescence phenotypes in high-yielding winter wheat, and their relationship to grain yield and grain protein concentration using high-throughput phenotyping techniques.Funct Plant Biol. 2014 Feb;41(3):227-235. doi: 10.1071/FP13221. Funct Plant Biol. 2014. PMID: 32480983
-
Applications of a Hyperspectral Imaging System Used to Estimate Wheat Grain Protein: A Review.Front Plant Sci. 2022 Apr 8;13:837200. doi: 10.3389/fpls.2022.837200. eCollection 2022. Front Plant Sci. 2022. PMID: 35463397 Free PMC article. Review.
Cited by
-
Retrieval of Hyperspectral Information from Multispectral Data for Perennial Ryegrass Biomass Estimation.Sensors (Basel). 2020 Dec 15;20(24):7192. doi: 10.3390/s20247192. Sensors (Basel). 2020. PMID: 33333952 Free PMC article.
-
Proximal and remote sensing in plant phenomics: 20 years of progress, challenges, and perspectives.Plant Commun. 2022 Nov 14;3(6):100344. doi: 10.1016/j.xplc.2022.100344. Epub 2022 Jun 2. Plant Commun. 2022. PMID: 35655429 Free PMC article. Review.
-
Reviewing the essential roles of remote phenotyping, GWAS and explainable AI in practical marker-assisted selection for drought-tolerant winter wheat breeding.Front Plant Sci. 2024 Apr 18;15:1319938. doi: 10.3389/fpls.2024.1319938. eCollection 2024. Front Plant Sci. 2024. PMID: 38699541 Free PMC article. Review.
-
Thermal imaging can reveal variation in stay-green functionality of wheat canopies under temperate conditions.Front Plant Sci. 2024 Jun 4;15:1335037. doi: 10.3389/fpls.2024.1335037. eCollection 2024. Front Plant Sci. 2024. PMID: 38895615 Free PMC article.
-
Water Stress Alters Physiological, Spectral, and Agronomic Indexes of Wheat Genotypes.Plants (Basel). 2023 Oct 14;12(20):3571. doi: 10.3390/plants12203571. Plants (Basel). 2023. PMID: 37896034 Free PMC article.
References
-
- Aasen H., Bolten A. (2018). Multi-temporal high-resolution imaging spectroscopy with hyperspectral 2D imagers – From theory to application. Remote Sens. Environ. 205, 374–389. 10.1016/j.rse.2017.10.043 - DOI
-
- Aasen H., Honkavaara E., Lucieer A., Zarco-Tejada P. J. (2018). Quantitative remote sensing at ultra-high resolution with uav spectroscopy: a review of sensor technology, measurement procedures, and data correction workflows. Remote Sens. 10, 1091. 10.3390/rs10071091 - DOI
-
- Asrar G., Fuchs M., Kanemasu E. T., Hatfield J. L. (1984). Estimating absorbed photosynthetic radiation and leaf area index from spectral reflectance in Wheat 1. Agron. J. 76, 300–306. 10.2134/agronj1984.00021962007600020029x - DOI
LinkOut - more resources
Full Text Sources