Brain-controlled modulation of spinal circuits improves recovery from spinal cord injury
- PMID: 30068906
- PMCID: PMC6070513
- DOI: 10.1038/s41467-018-05282-6
Brain-controlled modulation of spinal circuits improves recovery from spinal cord injury
Abstract
The delivery of brain-controlled neuromodulation therapies during motor rehabilitation may augment recovery from neurological disorders. To test this hypothesis, we conceived a brain-controlled neuromodulation therapy that combines the technical and practical features necessary to be deployed daily during gait rehabilitation. Rats received a severe spinal cord contusion that led to leg paralysis. We engineered a proportional brain-spine interface whereby cortical ensemble activity constantly determines the amplitude of spinal cord stimulation protocols promoting leg flexion during swing. After minimal calibration time and without prior training, this neural bypass enables paralyzed rats to walk overground and adjust foot clearance in order to climb a staircase. Compared to continuous spinal cord stimulation, brain-controlled stimulation accelerates and enhances the long-term recovery of locomotion. These results demonstrate the relevance of brain-controlled neuromodulation therapies to augment recovery from motor disorders, establishing important proofs-of-concept that warrant clinical studies.
Conflict of interest statement
S.M. and G.C. are founders and shareholders of GTX Medical, a company developing therapies in partial relationships with the topic of the submitted manuscript. M.B., S.M., and G.C. hold a patent on spatiotemporal neuromodulation algorithms (WO2015/063127). The remaining authors declare no competing interests.
Figures
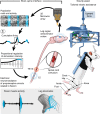
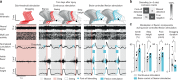
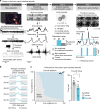
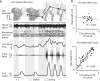
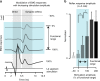
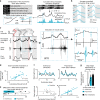
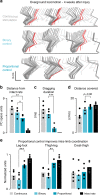
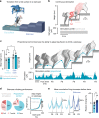
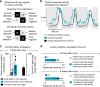
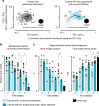
Similar articles
-
A brain-spine interface alleviating gait deficits after spinal cord injury in primates.Nature. 2016 Nov 10;539(7628):284-288. doi: 10.1038/nature20118. Nature. 2016. PMID: 27830790 Free PMC article.
-
Closed-loop neuromodulation of spinal sensorimotor circuits controls refined locomotion after complete spinal cord injury.Sci Transl Med. 2014 Sep 24;6(255):255ra133. doi: 10.1126/scitranslmed.3008325. Sci Transl Med. 2014. PMID: 25253676
-
Mechanisms Underlying the Neuromodulation of Spinal Circuits for Correcting Gait and Balance Deficits after Spinal Cord Injury.Neuron. 2016 Feb 17;89(4):814-28. doi: 10.1016/j.neuron.2016.01.009. Epub 2016 Feb 4. Neuron. 2016. PMID: 26853304
-
Plasticity of the spinal neural circuitry after injury.Annu Rev Neurosci. 2004;27:145-67. doi: 10.1146/annurev.neuro.27.070203.144308. Annu Rev Neurosci. 2004. PMID: 15217329 Review.
-
Electrical stimulation and motor recovery.Cell Transplant. 2015;24(3):429-46. doi: 10.3727/096368915X686904. Epub 2015 Feb 2. Cell Transplant. 2015. PMID: 25646771 Review.
Cited by
-
A new full closed-loop brain-machine interface approach based on neural activity: A study based on modeling and experimental studies.Heliyon. 2023 Feb 16;9(3):e13766. doi: 10.1016/j.heliyon.2023.e13766. eCollection 2023 Mar. Heliyon. 2023. PMID: 36851970 Free PMC article.
-
Insulin-like Growth Factor-1 Receptor Dictates Beneficial Effects of Treadmill Training by Regulating Survival and Migration of Neural Stem Cell Grafts in the Injured Spinal Cord.Exp Neurobiol. 2018 Dec;27(6):489-507. doi: 10.5607/en.2018.27.6.489. Epub 2018 Dec 28. Exp Neurobiol. 2018. PMID: 30636901 Free PMC article.
-
Axonal growth inhibitors and their receptors in spinal cord injury: from biology to clinical translation.Neural Regen Res. 2023 Dec;18(12):2573-2581. doi: 10.4103/1673-5374.373674. Neural Regen Res. 2023. PMID: 37449592 Free PMC article. Review.
-
A New Nonlinear Autoregressive Exogenous (NARX)-based Intra-spinal Stimulation Approach to Decode Brain Electrical Activity for Restoration of Leg Movement in Spinally-injured Rabbits.Basic Clin Neurosci. 2023 Jan-Feb;14(1):43-56. doi: 10.32598/bcn.2022.1840.1. Epub 2023 Jan 1. Basic Clin Neurosci. 2023. PMID: 37346873 Free PMC article.
-
Research hotspots and trends for axon regeneration (2000-2021): a bibliometric study and systematic review.Inflamm Regen. 2022 Dec 7;42(1):60. doi: 10.1186/s41232-022-00244-4. Inflamm Regen. 2022. PMID: 36476643 Free PMC article. Review.
References
Publication types
MeSH terms
Grants and funding
LinkOut - more resources
Full Text Sources
Other Literature Sources
Medical