Synchronous Spike Patterns in Macaque Motor Cortex during an Instructed-Delay Reach-to-Grasp Task
- PMID: 27511007
- PMCID: PMC4978798
- DOI: 10.1523/JNEUROSCI.4375-15.2016
Synchronous Spike Patterns in Macaque Motor Cortex during an Instructed-Delay Reach-to-Grasp Task
Abstract
The computational role of spike time synchronization at millisecond precision among neurons in the cerebral cortex is hotly debated. Studies performed on data of limited size provided experimental evidence that low-order correlations occur in relation to behavior. Advances in electrophysiological technology to record from hundreds of neurons simultaneously provide the opportunity to observe coordinated spiking activity of larger populations of cells. We recently published a method that combines data mining and statistical evaluation to search for significant patterns of synchronous spikes in massively parallel spike trains (Torre et al., 2013). The method solves the computational and multiple testing problems raised by the high dimensionality of the data. In the current study, we used our method on simultaneous recordings from two macaque monkeys engaged in an instructed-delay reach-to-grasp task to determine the emergence of spike synchronization in relation to behavior. We found a multitude of synchronous spike patterns aligned in both monkeys along a preferential mediolateral orientation in brain space. The occurrence of the patterns is highly specific to behavior, indicating that different behaviors are associated with the synchronization of different groups of neurons ("cell assemblies"). However, pooled patterns that overlap in neuronal composition exhibit no specificity, suggesting that exclusive cell assemblies become active during different behaviors, but can recruit partly identical neurons. These findings are consistent across multiple recording sessions analyzed across the two monkeys.
Significance statement: Neurons in the brain communicate via electrical impulses called spikes. How spikes are coordinated to process information is still largely unknown. Synchronous spikes are effective in triggering a spike emission in receiving neurons and have been shown to occur in relation to behavior in a number of studies on simultaneous recordings of few neurons. We recently published a method to extend this type of investigation to larger data. Here, we apply it to simultaneous recordings of hundreds of neurons from the motor cortex of macaque monkeys performing a motor task. Our analysis reveals groups of neurons selectively synchronizing their activity in relation to behavior, which sheds new light on the role of synchrony in information processing in the cerebral cortex.
Keywords: cell assemblies; massively parallel spike trains; motor cortex; spike synchrony; temporal coding.
Copyright © 2016 Torre, et al.
Figures
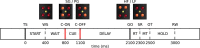
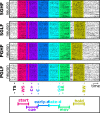
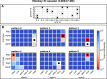
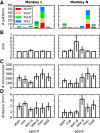
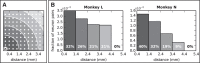
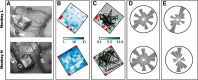
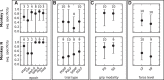
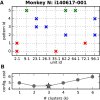
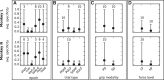
Similar articles
-
Synchronization in monkey motor cortex during a precision grip task. I. Task-dependent modulation in single-unit synchrony.J Neurophysiol. 2001 Feb;85(2):869-85. doi: 10.1152/jn.2001.85.2.869. J Neurophysiol. 2001. PMID: 11160519
-
Excess synchrony in motor cortical neurons provides redundant direction information with that from coarse temporal measures.J Neurophysiol. 2001 Oct;86(4):1700-16. doi: 10.1152/jn.2001.86.4.1700. J Neurophysiol. 2001. PMID: 11600633
-
Precise spatiotemporal repeating patterns in monkey primary and supplementary motor areas occur at chance levels.J Neurophysiol. 2000 Oct;84(4):1770-80. doi: 10.1152/jn.2000.84.4.1770. J Neurophysiol. 2000. PMID: 11024069
-
In Vivo Observations of Rapid Scattered Light Changes Associated with Neurophysiological Activity.In: Frostig RD, editor. In Vivo Optical Imaging of Brain Function. 2nd edition. Boca Raton (FL): CRC Press/Taylor & Francis; 2009. Chapter 5. In: Frostig RD, editor. In Vivo Optical Imaging of Brain Function. 2nd edition. Boca Raton (FL): CRC Press/Taylor & Francis; 2009. Chapter 5. PMID: 26844322 Free Books & Documents. Review.
-
Neural dynamics and information representation in microcircuits of motor cortex.Front Neural Circuits. 2013 May 3;7:85. doi: 10.3389/fncir.2013.00085. eCollection 2013. Front Neural Circuits. 2013. PMID: 23653596 Free PMC article. Review.
Cited by
-
Exploring retinal ganglion cells encoding to multi-modal stimulation using 3D microelectrodes arrays.Front Bioeng Biotechnol. 2023 Aug 1;11:1245082. doi: 10.3389/fbioe.2023.1245082. eCollection 2023. Front Bioeng Biotechnol. 2023. PMID: 37600306 Free PMC article.
-
Precise Spiking Motifs in Neurobiological and Neuromorphic Data.Brain Sci. 2022 Dec 29;13(1):68. doi: 10.3390/brainsci13010068. Brain Sci. 2022. PMID: 36672049 Free PMC article. Review.
-
Aberrant brain functional and structural developments in MECP2 duplication rats.Neurobiol Dis. 2022 Oct 15;173:105838. doi: 10.1016/j.nbd.2022.105838. Epub 2022 Aug 17. Neurobiol Dis. 2022. PMID: 35985556 Free PMC article.
-
Comparing Surrogates to Evaluate Precisely Timed Higher-Order Spike Correlations.eNeuro. 2022 Jun 9;9(3):ENEURO.0505-21.2022. doi: 10.1523/ENEURO.0505-21.2022. Print 2022 May-Jun. eNeuro. 2022. PMID: 35584914 Free PMC article.
-
1024-channel electrophysiological recordings in macaque V1 and V4 during resting state.Sci Data. 2022 Mar 11;9(1):77. doi: 10.1038/s41597-022-01180-1. Sci Data. 2022. PMID: 35277528 Free PMC article.
References
-
- Abeles M. Role of cortical neuron: integrator or coincidence detector? Isr J Med Sci. 1982;18:83–92. - PubMed
-
- Abeles M. Corticonics: neural circuits of the cerebral cortex. Cambridge: Cambridge University; 1991.
-
- Benjamini Y, Hochberg Y. Controlling the false discovery rate: a practical and powerful approach to multiple testing. J R Stat Soc Ser B. 1995;57:289–300.
Publication types
MeSH terms
LinkOut - more resources
Full Text Sources
Other Literature Sources