Parkin interacts with Ambra1 to induce mitophagy
- PMID: 21753002
- PMCID: PMC6623066
- DOI: 10.1523/JNEUROSCI.1917-11.2011
Parkin interacts with Ambra1 to induce mitophagy
Abstract
Mutations in the gene encoding Parkin are a major cause of recessive Parkinson's disease. Recent work has shown that Parkin translocates from the cytosol to depolarized mitochondria and induces their autophagic removal (mitophagy). However, the molecular mechanisms underlying Parkin-mediated mitophagy are poorly understood. Here, we investigated whether Parkin interacts with autophagy-regulating proteins. We purified Parkin and associated proteins from HEK293 cells using tandem affinity purification and identified the Parkin interactors using mass spectrometry. We identified the autophagy-promoting protein Ambra1 (activating molecule in Beclin1-regulated autophagy) as a Parkin interactor. Ambra1 activates autophagy in the CNS by stimulating the activity of the class III phosphatidylinositol 3-kinase (PI3K) complex that is essential for the formation of new phagophores. We found Ambra1, like Parkin, to be widely expressed in adult mouse brain, including midbrain dopaminergic neurons. Endogenous Parkin and Ambra1 coimmunoprecipitated from HEK293 cells, SH-SY5Y cells, and adult mouse brain. We found no evidence for ubiquitination of Ambra1 by Parkin. The interaction of endogenous Parkin and Ambra1 strongly increased during prolonged mitochondrial depolarization. Ambra1 was not required for Parkin translocation to depolarized mitochondria but was critically important for subsequent mitochondrial clearance. In particular, Ambra1 was recruited to perinuclear clusters of depolarized mitochondria and activated class III PI3K in their immediate vicinity. These data identify interaction of Parkin with Ambra1 as a key mechanism for induction of the final clearance step of Parkin-mediated mitophagy.
Figures
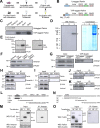
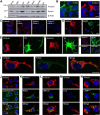
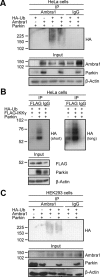
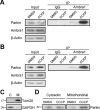
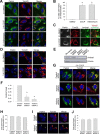
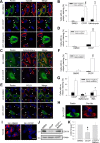
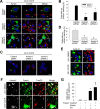
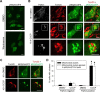
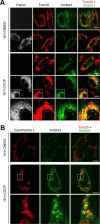
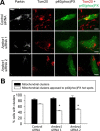
Similar articles
-
Ambra1: a Parkin-binding protein involved in mitophagy.Autophagy. 2011 Dec;7(12):1555-6. doi: 10.4161/auto.7.12.17893. Autophagy. 2011. PMID: 21921694 Free PMC article.
-
AMBRA1 is able to induce mitophagy via LC3 binding, regardless of PARKIN and p62/SQSTM1.Cell Death Differ. 2015 Mar;22(3):419-32. doi: 10.1038/cdd.2014.139. Epub 2014 Sep 12. Cell Death Differ. 2015. PMID: 25215947 Free PMC article.
-
miR-103a-3p regulates mitophagy in Parkinson's disease through Parkin/Ambra1 signaling.Pharmacol Res. 2020 Oct;160:105197. doi: 10.1016/j.phrs.2020.105197. Epub 2020 Sep 14. Pharmacol Res. 2020. PMID: 32942015
-
To eat or not to eat: neuronal metabolism, mitophagy, and Parkinson's disease.Antioxid Redox Signal. 2011 May 15;14(10):1979-87. doi: 10.1089/ars.2010.3763. Epub 2011 Mar 3. Antioxid Redox Signal. 2011. PMID: 21126205 Free PMC article. Review.
-
Parkin and mitophagy in cancer.Oncogene. 2017 Mar;36(10):1315-1327. doi: 10.1038/onc.2016.302. Epub 2016 Sep 5. Oncogene. 2017. PMID: 27593930 Review.
Cited by
-
From Brain to Muscle: The Role of Muscle Tissue in Neurodegenerative Disorders.Biology (Basel). 2024 Sep 12;13(9):719. doi: 10.3390/biology13090719. Biology (Basel). 2024. PMID: 39336146 Free PMC article. Review.
-
Emerging insights into pulmonary hypertension: the potential role of mitochondrial dysfunction and redox homeostasis.Mol Cell Biochem. 2024 Sep 10. doi: 10.1007/s11010-024-05096-9. Online ahead of print. Mol Cell Biochem. 2024. PMID: 39254871 Review.
-
Novel Insights into Parkin-Mediated Mitochondrial Dysfunction and "Mito-Inflammation" in α-Synuclein Toxicity. The Role of the cGAS-STING Signalling Pathway.J Inflamm Res. 2024 Jul 11;17:4549-4574. doi: 10.2147/JIR.S468609. eCollection 2024. J Inflamm Res. 2024. PMID: 39011416 Free PMC article. Review.
-
Liver Cell Mitophagy in Metabolic Dysfunction-Associated Steatotic Liver Disease and Liver Fibrosis.Antioxidants (Basel). 2024 Jun 15;13(6):729. doi: 10.3390/antiox13060729. Antioxidants (Basel). 2024. PMID: 38929168 Free PMC article. Review.
-
The Role and Mechanism of Ambra1-Mediated Mitophagy in TDCPP-Exposed Mouse Hippocampal Neurons.Neurochem Res. 2024 Sep;49(9):2453-2468. doi: 10.1007/s11064-024-04160-6. Epub 2024 Jun 8. Neurochem Res. 2024. PMID: 38850437
References
-
- Ahn S, Shenoy SK, Wei H, Lefkowitz RJ. Differential kinetic and spatial patterns of β-arrestin and G protein-mediated ERK activation by the angiotensin II receptor. J Biol Chem. 2004;279:35518–35525. - PubMed
-
- Clark IE, Dodson MW, Jiang C, Cao JH, Huh JR, Seol JH, Yoo SJ, Hay BA, Guo M. Drosophila pink1 is required for mitochondrial function and interacts genetically with parkin. Nature. 2006;441:1162–1166. - PubMed
-
- Collins MO, Choudhary JS. Mapping multiprotein complexes by affinity purification and mass spectrometry. Curr Opin Biotechnol. 2008;19:324–330. - PubMed
Publication types
MeSH terms
Substances
LinkOut - more resources
Full Text Sources
Molecular Biology Databases