Model-based influences on humans' choices and striatal prediction errors
- PMID: 21435563
- PMCID: PMC3077926
- DOI: 10.1016/j.neuron.2011.02.027
Model-based influences on humans' choices and striatal prediction errors
Abstract
The mesostriatal dopamine system is prominently implicated in model-free reinforcement learning, with fMRI BOLD signals in ventral striatum notably covarying with model-free prediction errors. However, latent learning and devaluation studies show that behavior also shows hallmarks of model-based planning, and the interaction between model-based and model-free values, prediction errors, and preferences is underexplored. We designed a multistep decision task in which model-based and model-free influences on human choice behavior could be distinguished. By showing that choices reflected both influences we could then test the purity of the ventral striatal BOLD signal as a model-free report. Contrary to expectations, the signal reflected both model-free and model-based predictions in proportions matching those that best explained choice behavior. These results challenge the notion of a separate model-free learner and suggest a more integrated computational architecture for high-level human decision-making.
Copyright © 2011 Elsevier Inc. All rights reserved.
Figures
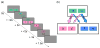
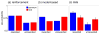
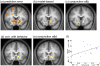
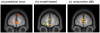
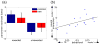
Similar articles
-
Striatal dopamine ramping may indicate flexible reinforcement learning with forgetting in the cortico-basal ganglia circuits.Front Neural Circuits. 2014 Apr 9;8:36. doi: 10.3389/fncir.2014.00036. eCollection 2014. Front Neural Circuits. 2014. PMID: 24782717 Free PMC article.
-
Hierarchical learning induces two simultaneous, but separable, prediction errors in human basal ganglia.J Neurosci. 2013 Mar 27;33(13):5797-805. doi: 10.1523/JNEUROSCI.5445-12.2013. J Neurosci. 2013. PMID: 23536092 Free PMC article.
-
Generalization of value in reinforcement learning by humans.Eur J Neurosci. 2012 Apr;35(7):1092-104. doi: 10.1111/j.1460-9568.2012.08017.x. Eur J Neurosci. 2012. PMID: 22487039 Free PMC article.
-
Functional properties of the basal ganglia's re-entrant loop architecture: selection and reinforcement.Neuroscience. 2011 Dec 15;198:138-51. doi: 10.1016/j.neuroscience.2011.07.060. Epub 2011 Jul 29. Neuroscience. 2011. PMID: 21821101 Review.
-
Navigating complex decision spaces: Problems and paradigms in sequential choice.Psychol Bull. 2014 Mar;140(2):466-86. doi: 10.1037/a0033455. Epub 2013 Jul 8. Psychol Bull. 2014. PMID: 23834192 Free PMC article. Review.
Cited by
-
Reinforcement learning approaches to hippocampus-dependent flexible spatial navigation.Brain Neurosci Adv. 2021 Apr 9;5:2398212820975634. doi: 10.1177/2398212820975634. eCollection 2021 Jan-Dec. Brain Neurosci Adv. 2021. PMID: 33954259 Free PMC article.
-
Protocol for a cluster randomised crossover pilot trial of Goal Management Training+ (GMT+) for methamphetamine use disorder.Contemp Clin Trials Commun. 2022 Aug 11;29:100969. doi: 10.1016/j.conctc.2022.100969. eCollection 2022 Oct. Contemp Clin Trials Commun. 2022. PMID: 36033363 Free PMC article.
-
Using data-driven model-brain mappings to constrain formal models of cognition.PLoS One. 2015 Mar 6;10(3):e0119673. doi: 10.1371/journal.pone.0119673. eCollection 2015. PLoS One. 2015. PMID: 25747601 Free PMC article.
-
Credit assignment in movement-dependent reinforcement learning.Proc Natl Acad Sci U S A. 2016 Jun 14;113(24):6797-802. doi: 10.1073/pnas.1523669113. Epub 2016 May 31. Proc Natl Acad Sci U S A. 2016. PMID: 27247404 Free PMC article. Clinical Trial.
-
Grid-like entorhinal representation of an abstract value space during prospective decision making.Nat Commun. 2024 Feb 9;15(1):1198. doi: 10.1038/s41467-024-45127-z. Nat Commun. 2024. PMID: 38336756 Free PMC article.
References
-
- Adams C. Variations in the sensitivity of instrumental responding to reinforcer devaluation. The Quarterly Journal of Experimental Psychology Section B. 1982;34:77–98.
-
- Balleine BW, Daw ND, O’Doherty JP. Multiple forms of value learning and the function of dopamine. In: Glimcher PW, Camerer C, Poldrack RA, Fehr E, editors. Neuroeconomics: Decision Making and the Brain. Academic Press; 2008.
-
- Ballmaier M, Toga A, Siddarth P, Blanton R, Levitt J, Lee M, Caplan R. Thought disorder and nucleus accumbens in childhood: a structural MRI study. Psychiatry Res. 2004;130:43–55. - PubMed
-
- Barto A, Sutton R, Anderson C. Neuronlike adaptive elements that can solve difficult learning control problems. IEEE Transactions on systems, man, and cybernetics. 1983;13:834–846.
Publication types
MeSH terms
Substances
Grants and funding
LinkOut - more resources
Full Text Sources
Other Literature Sources