Participation of autophagy in storage of lysosomes in neurons from mouse models of neuronal ceroid-lipofuscinoses (Batten disease)
- PMID: 16314482
- PMCID: PMC1613187
- DOI: 10.1016/S0002-9440(10)61253-9
Participation of autophagy in storage of lysosomes in neurons from mouse models of neuronal ceroid-lipofuscinoses (Batten disease)
Abstract
In cathepsin D-deficient (CD-/-) and cathepsins B and L double-deficient (CB-/-CL-/-) mice, abnormal vacuolar structures accumulate in neurons of the brains. Many of these structures resemble autophagosomes in which part of the cytoplasm is retained but their precise nature and biogenesis remain unknown. We show here how autophagy contributes to the accumulation of these vacuolar structures in neurons deficient in cathepsin D or both cathepsins B and L by demonstrating an increased conversion of the molecular form of MAP1-LC3 for autophagosome formation from the cytosolic form (LC3-I) to the membrane-bound form (LC3-II). In both CD-/- and CB-/-CL-/- mouse brains, the membrane-bound LC3-II form predominated whereas MAP1-LC3 signals accumulated in granular structures located in neuronal perikarya and axons of these mutant brains and were localized to the membranes of autophagosomes, evidenced by immunofluorescence microscopy and freeze-fracture-replica immunoelectron microscopy. Moreover, as in CD-/- neurons, autofluorescence and subunit c of mitochondrial ATP synthase accumulated in CB-/-CL-/- neurons. This suggests that not only CD-/- but also CB-/-CL-/- mice could be useful animal models for neuronal ceroid-lipofuscinosis/Batten disease. These data strongly argue for a major involvement of autophagy in the pathogenesis of Batten disease/lysosomal storage disorders.
Figures
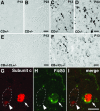
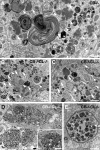
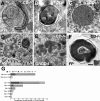
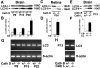
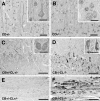
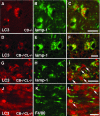
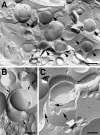
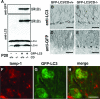
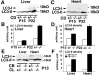
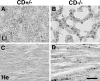
Comment in
-
Cathepsin deficiency as a model for neuronal ceroid lipofuscinoses.Am J Pathol. 2005 Dec;167(6):1473-6. doi: 10.1016/S0002-9440(10)61233-3. Am J Pathol. 2005. PMID: 16314462 Free PMC article. Review. No abstract available.
Similar articles
-
Cathepsin D deficiency induces lysosomal storage with ceroid lipofuscin in mouse CNS neurons.J Neurosci. 2000 Sep 15;20(18):6898-906. doi: 10.1523/JNEUROSCI.20-18-06898.2000. J Neurosci. 2000. PMID: 10995834 Free PMC article.
-
Lysosomal dysfunction and impaired autophagy in a novel mouse model deficient for the lysosomal membrane protein Cln7.Hum Mol Genet. 2016 Feb 15;25(4):777-91. doi: 10.1093/hmg/ddv615. Epub 2015 Dec 17. Hum Mol Genet. 2016. PMID: 26681805
-
Cathepsin D deficiency induces persistent neurodegeneration in the absence of Bax-dependent apoptosis.J Neurosci. 2007 Feb 21;27(8):2081-90. doi: 10.1523/JNEUROSCI.5577-06.2007. J Neurosci. 2007. PMID: 17314303 Free PMC article.
-
Cathepsin D deficiency and NCL/Batten disease: there's more to death than apoptosis.Autophagy. 2007 Sep-Oct;3(5):474-6. doi: 10.4161/auto.4341. Epub 2007 Apr 25. Autophagy. 2007. PMID: 17495518 Review.
-
NCL disease mechanisms.Biochim Biophys Acta. 2013 Nov;1832(11):1882-93. doi: 10.1016/j.bbadis.2013.05.014. Epub 2013 May 23. Biochim Biophys Acta. 2013. PMID: 23707513 Review.
Cited by
-
Cathepsin D overexpression in the nervous system rescues lethality and Aβ42 accumulation of cathepsin D systemic knockout in vivo.Acta Pharm Sin B. 2023 Oct;13(10):4172-4184. doi: 10.1016/j.apsb.2023.07.015. Epub 2023 Jul 20. Acta Pharm Sin B. 2023. PMID: 37799377 Free PMC article.
-
A Homozygous MAN2B1 Missense Mutation in a Doberman Pinscher Dog with Neurodegeneration, Cytoplasmic Vacuoles, Autofluorescent Storage Granules, and an α-Mannosidase Deficiency.Genes (Basel). 2023 Aug 31;14(9):1746. doi: 10.3390/genes14091746. Genes (Basel). 2023. PMID: 37761886 Free PMC article.
-
Reciprocal effects of alpha-synuclein aggregation and lysosomal homeostasis in synucleinopathy models.Transl Neurodegener. 2023 Jun 13;12(1):31. doi: 10.1186/s40035-023-00363-z. Transl Neurodegener. 2023. PMID: 37312133 Free PMC article.
-
Extracellular Vesicles Released by Genetically Modified Macrophages Activate Autophagy and Produce Potent Neuroprotection in Mouse Model of Lysosomal Storage Disorder, Batten Disease.Cells. 2023 May 29;12(11):1497. doi: 10.3390/cells12111497. Cells. 2023. PMID: 37296618 Free PMC article.
-
Cathepsins Trigger Cell Death and Regulate Radioresistance in Glioblastoma.Cells. 2022 Dec 17;11(24):4108. doi: 10.3390/cells11244108. Cells. 2022. PMID: 36552871 Free PMC article.
References
-
- Vittorini S, Paradiso C, Donati A, Cavallini G, Masini M, Gori Z, Pollera M, Bergamini E. The age-related accumulation of protein carbonyl in rat liver correlates with the age-related decline in liver proteolytic activities. J Gerontol A Biol Sci Med Sci. 1999;54:B318–B323. - PubMed
-
- Larsen KE, Sulzer D. Autophagy in neurons: a review. Histol Histopathol. 2002;17:897–908. - PubMed
-
- Uchiyama Y. Autophagic cell death and its execution by lysosomal cathepsins. Arch Histol Cytol. 2001;64:233–246. - PubMed
-
- Yuan J, Lipinski M, Degterev A. Diversity in the mechanisms of neuronal cell death. Neuron. 2003;40:401–413. - PubMed
Publication types
MeSH terms
Substances
LinkOut - more resources
Full Text Sources
Other Literature Sources
Molecular Biology Databases
Research Materials