Orientation selectivity and the arrangement of horizontal connections in tree shrew striate cortex
- PMID: 9045738
- PMCID: PMC6793759
- DOI: 10.1523/JNEUROSCI.17-06-02112.1997
Orientation selectivity and the arrangement of horizontal connections in tree shrew striate cortex
Abstract
Horizontal connections, formed primarily by the axon collaterals of pyramidal neurons in layer 2/3 of visual cortex, extend for millimeters parallel to the cortical surface and form patchy terminations. Previous studies have provided evidence that the patches formed by horizontal connections exhibit modular specificity, preferentially linking columns of neurons with similar response characteristics, such as preferred orientation. The issue of how these connections are distributed with respect to the topographic map of visual space, however, has not been resolved. Here we combine optical imaging of intrinsic signals with small extracellular injections of biocytin to assess quantitatively the specificity of horizontal connections with respect to both the map of orientation preference and the map of visual space in tree shrew V1. Our results indicate that horizontal connections outside a radius of 500 microm from the injection site exhibit not only modular specificity, but also specificity for axis of projection. Labeled axons extend for longer distances, and give off more terminal boutons, along an axis in the map of visual space that corresponds to the preferred orientation of the injection site. Inside of 500 microm, the pattern of connections is much less specific, with boutons found along every axis, contacting sites with a wide range of preferred orientations. The system of long-range horizontal connections can be summarized as preferentially linking neurons with co-oriented, co-axially aligned receptive fields. These observations suggest specific ways that horizontal circuits contribute to the response properties of layer 2/3 neurons and to mechanisms of visual perception.
Figures
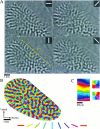
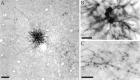
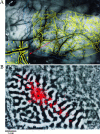
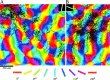
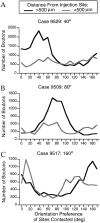
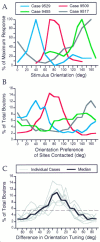
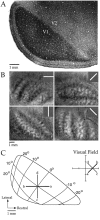
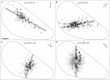
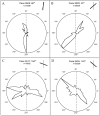
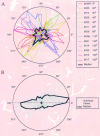
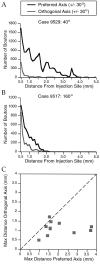
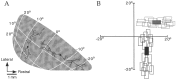
Similar articles
-
The functional organization of local circuits in visual cortex: insights from the study of tree shrew striate cortex.Cereb Cortex. 1996 May-Jun;6(3):329-41. doi: 10.1093/cercor/6.3.329. Cereb Cortex. 1996. PMID: 8670661 Review.
-
The contribution of vertical and horizontal connections to the receptive field center and surround in V1.Neural Netw. 2004 Jun-Jul;17(5-6):681-93. doi: 10.1016/j.neunet.2004.05.002. Neural Netw. 2004. PMID: 15288892 Review.
-
A morphological basis for orientation tuning in primary visual cortex.Nat Neurosci. 2004 Aug;7(8):872-9. doi: 10.1038/nn1287. Epub 2004 Jul 18. Nat Neurosci. 2004. PMID: 15258585
-
Topographic organization of the orientation column system in the striate cortex of the tree shrew (Tupaia glis). II. Deoxyglucose mapping.J Comp Neurol. 1980 Aug 1;192(3):549-66. doi: 10.1002/cne.901920312. J Comp Neurol. 1980. PMID: 7419744
-
Relationship between intrinsic connections and functional architecture revealed by optical imaging and in vivo targeted biocytin injections in primate striate cortex.Proc Natl Acad Sci U S A. 1993 Nov 15;90(22):10469-73. doi: 10.1073/pnas.90.22.10469. Proc Natl Acad Sci U S A. 1993. PMID: 8248133 Free PMC article.
Cited by
-
Organization of Anti-Phase Synchronization Pattern in Neural Networks: What are the Key Factors?Front Syst Neurosci. 2011 Dec 7;5:100. doi: 10.3389/fnsys.2011.00100. eCollection 2011. Front Syst Neurosci. 2011. PMID: 22232576 Free PMC article.
-
Correlated variability and its attentional modulation depend on anatomical connectivity.Proc Natl Acad Sci U S A. 2024 Aug 27;121(35):e2318841121. doi: 10.1073/pnas.2318841121. Epub 2024 Aug 22. Proc Natl Acad Sci U S A. 2024. PMID: 39172780
-
Beyond Columnar Organization: Cell Type- and Target Layer-Specific Principles of Horizontal Axon Projection Patterns in Rat Vibrissal Cortex.Cereb Cortex. 2015 Nov;25(11):4450-68. doi: 10.1093/cercor/bhv053. Epub 2015 Apr 1. Cereb Cortex. 2015. PMID: 25838038 Free PMC article.
-
Correlations and Neuronal Population Information.Annu Rev Neurosci. 2016 Jul 8;39:237-56. doi: 10.1146/annurev-neuro-070815-013851. Epub 2016 Apr 21. Annu Rev Neurosci. 2016. PMID: 27145916 Free PMC article. Review.
-
Collective Activity of Many Bistable Assemblies Reproduces Characteristic Dynamics of Multistable Perception.J Neurosci. 2016 Jun 29;36(26):6957-72. doi: 10.1523/JNEUROSCI.4626-15.2016. J Neurosci. 2016. PMID: 27358454 Free PMC article.
References
-
- Albus K, Wahle P. The topography of tangential inhibitory connections in the postnatally developing and mature striate cortex of the cat. Eur J Neurosci. 1994;6:779–792. - PubMed
-
- Albus K, Wahle P, Lubke J, Matute C. The contribution of GABA-ergic neurons to horizontal intrinsic connections in upper layers of the cat’s striate cortex. Exp Brain Res. 1991;85:235–239. - PubMed
-
- Amir Y, Harel M, Malach R. Cortical hierarchy reflected in the organization of intrinsic connections in macaque monkey visual cortex. J Comp Neurol. 1993;334:19–46. - PubMed
-
- Blasdel GG, Yoshioka T, Levitt JB, Lund JS. Correlation between patterns of lateral connectivity and patterns of orientation preference in monkey striate cortex. Soc Neurosci Abstr. 1992;18:389.
Publication types
MeSH terms
Grants and funding
LinkOut - more resources
Full Text Sources
Other Literature Sources