lifex-ep: a robust and efficient software for cardiac electrophysiology simulations
- PMID: 37828428
- PMCID: PMC10571323
- DOI: 10.1186/s12859-023-05513-8
lifex-ep: a robust and efficient software for cardiac electrophysiology simulations
Abstract
Background: Simulating the cardiac function requires the numerical solution of multi-physics and multi-scale mathematical models. This underscores the need for streamlined, accurate, and high-performance computational tools. Despite the dedicated endeavors of various research teams, comprehensive and user-friendly software programs for cardiac simulations, capable of accurately replicating both normal and pathological conditions, are still in the process of achieving full maturity within the scientific community.
Results: This work introduces [Formula: see text]-ep, a publicly available software for numerical simulations of the electrophysiology activity of the cardiac muscle, under both normal and pathological conditions. [Formula: see text]-ep employs the monodomain equation to model the heart's electrical activity. It incorporates both phenomenological and second-generation ionic models. These models are discretized using the Finite Element method on tetrahedral or hexahedral meshes. Additionally, [Formula: see text]-ep integrates the generation of myocardial fibers based on Laplace-Dirichlet Rule-Based Methods, previously released in Africa et al., 2023, within [Formula: see text]-fiber. As an alternative, users can also choose to import myofibers from a file. This paper provides a concise overview of the mathematical models and numerical methods underlying [Formula: see text]-ep, along with comprehensive implementation details and instructions for users. [Formula: see text]-ep features exceptional parallel speedup, scaling efficiently when using up to thousands of cores, and its implementation has been verified against an established benchmark problem for computational electrophysiology. We showcase the key features of [Formula: see text]-ep through various idealized and realistic simulations conducted in both normal and pathological scenarios. Furthermore, the software offers a user-friendly and flexible interface, simplifying the setup of simulations using self-documenting parameter files.
Conclusions: [Formula: see text]-ep provides easy access to cardiac electrophysiology simulations for a wide user community. It offers a computational tool that integrates models and accurate methods for simulating cardiac electrophysiology within a high-performance framework, while maintaining a user-friendly interface. [Formula: see text]-ep represents a valuable tool for conducting in silico patient-specific simulations.
Keywords: Cardiac electrophysiology; Computational cardiology; Finite element method; High-performance computing; Mathematical modeling.
© 2023. BioMed Central Ltd., part of Springer Nature.
Conflict of interest statement
The authors declare that they have no competing interests.
Figures
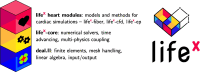
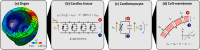
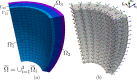
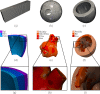
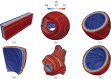
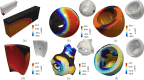
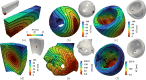
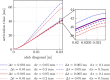
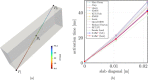
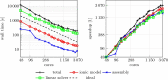
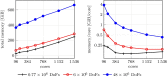
Similar articles
-
lifex-fiber: an open tool for myofibers generation in cardiac computational models.BMC Bioinformatics. 2023 Apr 12;24(1):143. doi: 10.1186/s12859-023-05260-w. BMC Bioinformatics. 2023. PMID: 37046208 Free PMC article.
-
An inner-outer subcycling algorithm for parallel cardiac electrophysiology simulations.Int J Numer Method Biomed Eng. 2023 Mar;39(3):e3677. doi: 10.1002/cnm.3677. Epub 2023 Jan 9. Int J Numer Method Biomed Eng. 2023. PMID: 36573938
-
Macromolecular crowding: chemistry and physics meet biology (Ascona, Switzerland, 10-14 June 2012).Phys Biol. 2013 Aug;10(4):040301. doi: 10.1088/1478-3975/10/4/040301. Epub 2013 Aug 2. Phys Biol. 2013. PMID: 23912807
-
The GLEaMviz computational tool, a publicly available software to explore realistic epidemic spreading scenarios at the global scale.BMC Infect Dis. 2011 Feb 2;11:37. doi: 10.1186/1471-2334-11-37. BMC Infect Dis. 2011. PMID: 21288355 Free PMC article. Review.
-
New computational methods in tsunami science.Philos Trans A Math Phys Eng Sci. 2015 Oct 28;373(2053):20140382. doi: 10.1098/rsta.2014.0382. Philos Trans A Math Phys Eng Sci. 2015. PMID: 26392612 Review.
Cited by
-
The Ithildin library for efficient numerical solution of anisotropic reaction-diffusion problems in excitable media.PLoS One. 2024 Sep 19;19(9):e0303674. doi: 10.1371/journal.pone.0303674. eCollection 2024. PLoS One. 2024. PMID: 39298417 Free PMC article.
-
The role of computational methods in cardiovascular medicine: a narrative review.Transl Pediatr. 2024 Jan 29;13(1):146-163. doi: 10.21037/tp-23-184. Epub 2024 Jan 24. Transl Pediatr. 2024. PMID: 38323181 Free PMC article. Review.
References
-
- Tortora GJ, Derrickson BH. Principles of anatomy and physiology. London: Wiley; 2008.
-
- Katz AM. Physiology of the heart. Wilkins: Lippincott Williams; 2010.
-
- Klabunde R. Cardiovascular physiology concepts. Wilkins: Lippincott Williams; 2011.
-
- Harrington RA, Narula J, Eapen ZJ. Hurst’s the Heart. MacGraw-Hill 2011.
-
- Quarteroni A, Dede’ L, Manzoni A, Vergara C. Mathematical modelling of the human cardiovascular system: data, numerical approximation. Clinical Applications: Cambridge University Press; 2019.
MeSH terms
Grants and funding
LinkOut - more resources
Full Text Sources
Research Materials