The Optimized Delivery of Triterpenes by Liposomal Nanoformulations: Overcoming the Challenges
- PMID: 35163063
- PMCID: PMC8835305
- DOI: 10.3390/ijms23031140
The Optimized Delivery of Triterpenes by Liposomal Nanoformulations: Overcoming the Challenges
Abstract
The last decade has witnessed a sustained increase in the research development of modern-day chemo-therapeutics, especially for those used for high mortality rate pathologies. However, the therapeutic landscape is continuously changing as a result of the currently existing toxic side effects induced by a substantial range of drug classes. One growing research direction driven to mitigate such inconveniences has converged towards the study of natural molecules for their promising therapeutic potential. Triterpenes are one such class of compounds, intensively investigated for their therapeutic versatility. Although the pharmacological effects reported for several representatives of this class has come as a well-deserved encouragement, the pharmacokinetic profile of these molecules has turned out to be an unwelcomed disappointment. Nevertheless, the light at the end of the tunnel arrived with the development of nanotechnology, more specifically, the use of liposomes as drug delivery systems. Liposomes are easily synthesizable phospholipid-based vesicles, with highly tunable surfaces, that have the ability to transport both hydrophilic and lipophilic structures ensuring superior drug bioavailability at the action site as well as an increased selectivity. This study aims to report the results related to the development of different types of liposomes, used as targeted vectors for the delivery of various triterpenes of high pharmacological interest.
Keywords: liposomes; nano-therapy; nanocarriers; targeted delivery; triterpenes.
Conflict of interest statement
The authors declare no conflict of interest.
Figures
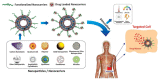
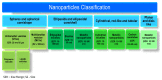
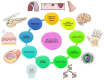
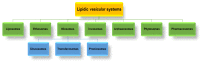
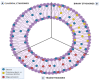
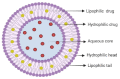
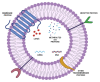

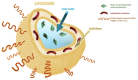
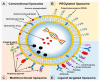
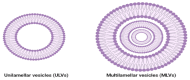
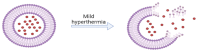
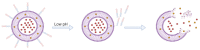
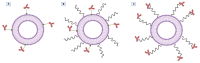
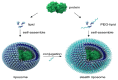
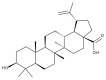
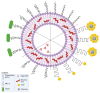
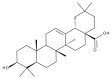
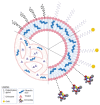
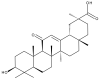
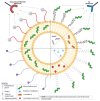
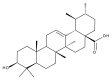
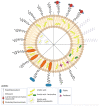
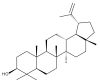
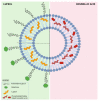
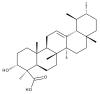
Similar articles
-
Enhanced Pharmacokinetics of Celastrol via Long-Circulating Liposomal Delivery for Intravenous Administration.Int J Nanomedicine. 2024 Jun 11;19:5707-5718. doi: 10.2147/IJN.S461624. eCollection 2024. Int J Nanomedicine. 2024. PMID: 38882540 Free PMC article.
-
Formulation and evaluation of celastrol-loaded liposomes.Molecules. 2011 Sep 13;16(9):7880-92. doi: 10.3390/molecules16097880. Molecules. 2011. PMID: 22143548 Free PMC article.
-
Preparation, characterization, and assessment of the antiglioma effects of liposomal celastrol.Anticancer Drugs. 2012 Jun;23(5):515-24. doi: 10.1097/CAD.0b013e3283514b68. Anticancer Drugs. 2012. PMID: 22343423
-
Liposomes in biology and medicine.Adv Exp Med Biol. 2007;620:117-28. doi: 10.1007/978-0-387-76713-0_9. Adv Exp Med Biol. 2007. PMID: 18217339 Review.
-
Potential use of nanocarriers with pentacyclic triterpenes in cancer treatments.Nanomedicine (Lond). 2016 Dec;11(23):3139-3156. doi: 10.2217/nnm-2016-0251. Epub 2016 Nov 4. Nanomedicine (Lond). 2016. PMID: 27809705 Review.
Cited by
-
Tuning of the Anti-Breast Cancer Activity of Betulinic Acid via Its Conversion to Ionic Liquids.Pharmaceutics. 2024 Apr 3;16(4):496. doi: 10.3390/pharmaceutics16040496. Pharmaceutics. 2024. PMID: 38675157 Free PMC article.
-
Triterpenes Drug Delivery Systems, a Modern Approach for Arthritis Targeted Therapy.Pharmaceuticals (Basel). 2023 Dec 28;17(1):54. doi: 10.3390/ph17010054. Pharmaceuticals (Basel). 2023. PMID: 38256888 Free PMC article. Review.
-
Ethanolic Extract Propolis-Loaded Niosomes Diminish Phospholipase B1, Biofilm Formation, and Intracellular Replication of Cryptococcus neoformans in Macrophages.Molecules. 2023 Aug 24;28(17):6224. doi: 10.3390/molecules28176224. Molecules. 2023. PMID: 37687052 Free PMC article.
-
Extracellular Vesicles Released by Genetically Modified Macrophages Activate Autophagy and Produce Potent Neuroprotection in Mouse Model of Lysosomal Storage Disorder, Batten Disease.Cells. 2023 May 29;12(11):1497. doi: 10.3390/cells12111497. Cells. 2023. PMID: 37296618 Free PMC article.
-
Semisynthetic Derivatives of Pentacyclic Triterpenes Bearing Heterocyclic Moieties with Therapeutic Potential.Molecules. 2022 Oct 3;27(19):6552. doi: 10.3390/molecules27196552. Molecules. 2022. PMID: 36235089 Free PMC article. Review.
References
Publication types
MeSH terms
Substances
Grants and funding
LinkOut - more resources
Full Text Sources