CALIPSO Lidar Calibration at 532 nm: Version 4 Nighttime Algorithm
- PMID: 33479568
- PMCID: PMC7816828
- DOI: 10.5194/amt-11-1459-2018
CALIPSO Lidar Calibration at 532 nm: Version 4 Nighttime Algorithm
Abstract
Data products from the Cloud-Aerosol Lidar with Orthogonal Polarization (CALIOP) on board Cloud-Aerosol Lidar and Infrared Pathfinder Satellite Observations (CALIPSO) were recently updated following the implementation of new (version 4) calibration algorithms for all of the level 1 attenuated backscatter measurements. In this work we present the motivation for and the implementation of the version 4 nighttime 532 nm parallel channel calibration. The nighttime 532 nm calibration is the most fundamental calibration of CALIOP data, since all of CALIOP's other radiometric calibration procedures - i.e., the 532 nm daytime calibration and the 1064 nm calibrations during both nighttime and daytime - depend either directly or indirectly on the 532 nm nighttime calibration. The accuracy of the 532 nm nighttime calibration has been significantly improved by raising the molecular normalization altitude from 30-34 km to 36-39 km to substantially reduce stratospheric aerosol contamination. Due to the greatly reduced molecular number density and consequently reduced signal-to-noise ratio (SNR) at these higher altitudes, the signal is now averaged over a larger number of samples using data from multiple adjacent granules. As well, an enhanced strategy for filtering the radiation-induced noise from high energy particles was adopted. Further, the meteorological model used in the earlier versions has been replaced by the improved MERRA-2 model. An aerosol scattering ratio of 1.01 ± 0.01 is now explicitly used for the calibration altitude. These modifications lead to globally revised calibration coefficients which are, on average, 2-3% lower than in previous data releases. Further, the new calibration procedure is shown to eliminate biases at high altitudes that were present in earlier versions and consequently leads to an improved representation of stratospheric aerosols. Validation results using airborne lidar measurements are also presented. Biases relative to collocated measurements acquired by the Langley Research Center (LaRC) airborne high spectral resolution lidar (HSRL) are reduced from 3.6% ± 2.2% in the version 3 data set to 1.6% ± 2.4 % in the version 4 release.
Figures
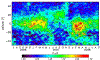
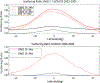
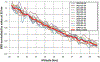
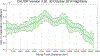
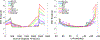
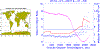
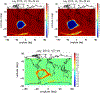
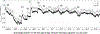
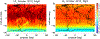
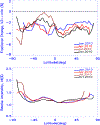
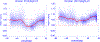
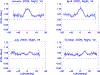
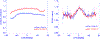
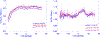
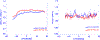
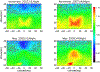
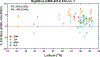
Similar articles
-
Cloud Aerosol Transport System (CATS) 1064 nm Calibration and Validation.Atmos Meas Tech. 2019 Nov 28;12(11):6241-6258. doi: 10.5194/amt-12-6241-2019. Atmos Meas Tech. 2019. PMID: 33414857 Free PMC article.
-
The CALIPSO Version 4 Automated Aerosol Classification and Lidar Ratio Selection Algorithm.Atmos Meas Tech. 2018;11(11):6107-6135. doi: 10.5194/amt-11-6107-2018. Atmos Meas Tech. 2018. PMID: 31921372 Free PMC article.
-
Depolarization ratio and attenuated backscatter for nine cloud types: analyses based on collocated CALIPSO lidar and MODIS measurements.Opt Express. 2008 Mar 17;16(6):3931-48. doi: 10.1364/oe.16.003931. Opt Express. 2008. PMID: 18542490
-
Performance estimation of space-borne high-spectral-resolution lidar for cloud and aerosol optical properties at 532 nm.Opt Express. 2019 Apr 15;27(8):A481-A494. doi: 10.1364/OE.27.00A481. Opt Express. 2019. PMID: 31052898
-
Comparisons of aerosol backscatter using satellite and ground lidars: implications for calibrating and validating spaceborne lidar.Sci Rep. 2017 Feb 15;7:42337. doi: 10.1038/srep42337. Sci Rep. 2017. PMID: 28198389 Free PMC article.
Cited by
-
Decreased dust particles amplify the cloud cooling effect by regulating cloud ice formation over the Tibetan Plateau.Sci Adv. 2024 Sep 13;10(37):eado0885. doi: 10.1126/sciadv.ado0885. Epub 2024 Sep 13. Sci Adv. 2024. PMID: 39270018 Free PMC article.
-
Black carbon aerosol number and mass concentration measurements by picosecond short-range elastic backscatter lidar.Sci Rep. 2022 May 19;12(1):8443. doi: 10.1038/s41598-022-11954-7. Sci Rep. 2022. PMID: 35589746 Free PMC article.
-
Cloud Aerosol Transport System (CATS) 1064 nm Calibration and Validation.Atmos Meas Tech. 2019 Nov 28;12(11):6241-6258. doi: 10.5194/amt-12-6241-2019. Atmos Meas Tech. 2019. PMID: 33414857 Free PMC article.
-
The CALIPSO Version 4 Automated Aerosol Classification and Lidar Ratio Selection Algorithm.Atmos Meas Tech. 2018;11(11):6107-6135. doi: 10.5194/amt-11-6107-2018. Atmos Meas Tech. 2018. PMID: 31921372 Free PMC article.
-
Intercomparison of in-situ aircraft and satellite aerosol measurements in the stratosphere.Sci Rep. 2019 Oct 30;9(1):15576. doi: 10.1038/s41598-019-52089-6. Sci Rep. 2019. PMID: 31666595 Free PMC article.
References
-
- Behrenfeld MJ, Hu Y, O’Malley RT, Boss ES, Hostetler CA, Siegel DA, Sarmiento JL, Schulien J, Hair JW, Lu X, Rodier S and Scarino AJ: Annual boom - bust cycles of polar phytoplankton biomass revealed by space-based lidar, Nature Geosci, 10, 118–122, doi:10.1038/ngeo2861, 2017. - DOI
-
- Bertaux JL, Kyrola E, Fussen D, Hauchecorne A, Dalaudier F, Sofieva V, Tamminen J, Vanhellemont F, Fanton d’Andon O, Barrot G, Mangin A, Blanot L, Lebrun JC, Perot K, Fehr T, Saavedra L, Leppelmeier GW, Fraisse R: Global ozone monitoring by occultation of stars: An overview of GOMOS measurements on ENVISAT, Atmos. Chem. Phys, 10, 12091–12148, 2010.
-
- Damadeo RP, Zawodny JM, Thomason LW and Iyer N: SAGE version 7.0 algorithm: Application to SAGE II, Atmos. Meas. Tech, 6, 3539–3561, 2013.
-
- Domingos J, Jault D, Pais MA and Mandea M: The South Atlantic Anomaly throughout the solar cycle, Earth Planet. Sc. Lett, 473, 154–163, doi:10.1016/j.epsl.2017.06.004, 2017. - DOI
-
- Garnier A, Scott NA, Pelon J, Armante R, Crépeau L, Six B, and Pascal N: Long term assessment of the CALIPSO Imaging Infrared Radiometer (IIR) calibration and stability through comparisons with MODIS/Aqua and SEVIRI/Meteosat, Atmos. Meas. Tech, 10, 1403–1424, doi:10.5194/amt-10-1403-2017, 2017. - DOI
Grants and funding
LinkOut - more resources
Full Text Sources
Other Literature Sources
Research Materials