The Cellular and Mechanical Basis for Response Characteristics of Identified Primary Afferents in the Rat Vibrissal System
- PMID: 32004452
- PMCID: PMC10623402
- DOI: 10.1016/j.cub.2019.12.068
The Cellular and Mechanical Basis for Response Characteristics of Identified Primary Afferents in the Rat Vibrissal System
Abstract
Compared to our understanding of the response properties of receptors in the auditory and visual systems, we have only a limited understanding of the mechanoreceptor responses that underlie tactile sensation. Here, we exploit the stereotyped morphology of the rat vibrissal (whisker) array to investigate coding and transduction properties of identified primary tactile afferents. We performed in vivo intra-axonal recording and labeling experiments to quantify response characteristics of four different types of identified mechanoreceptors in the vibrissal follicle: ring-sinus Merkel; lanceolate; clublike; and rete-ridge collar Merkel. Of these types, only ring-sinus Merkel endings exhibited slowly adapting properties. A weak inverse relationship between response magnitude and onset response latency was found across all types. All afferents exhibited strong "angular tuning," i.e., their response magnitude and latency depended on the whisker's deflection angle. Although previous studies suggested that this tuning should be aligned with the angular location of the mechanoreceptor in the follicle, such alignment was observed only for Merkel afferents; angular tuning of the other afferent types showed no clear alignment with mechanoreceptor location. Biomechanical modeling suggested that this tuning difference might be explained by mechanoreceptors' differential sensitivity to the force directed along the whisker length. Electron microscopic investigations of Merkel endings and lanceolate endings at the level of the ring sinus revealed unique anatomical features that may promote these differential sensitivities. The present study systematically integrates biomechanical principles with the anatomical and morphological characterization of primary afferent endings to describe the physical and cellular processing that shapes the neural representation of touch.
Keywords: 3D reconstruction; active sensing; firing properties; ganglion; in vivo recording; peripheral system; piezoelectric stimulator; rodent; single-cell labeling; trigeminal.
Copyright © 2019 The Authors. Published by Elsevier Inc. All rights reserved.
Conflict of interest statement
Declaration of Interests The authors declare no competing interests.
Figures
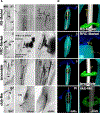
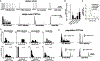
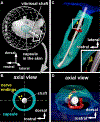
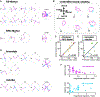
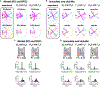
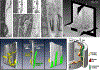
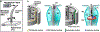
Comment in
-
Somatosensation: The Cellular and Physical Basis of Tactile Experience.Curr Biol. 2020 Mar 9;30(5):R215-R217. doi: 10.1016/j.cub.2020.01.020. Curr Biol. 2020. PMID: 32155422
Similar articles
-
Whisker Vibrations and the Activity of Trigeminal Primary Afferents in Response to Airflow.J Neurosci. 2019 Jul 24;39(30):5881-5896. doi: 10.1523/JNEUROSCI.2971-18.2019. Epub 2019 May 16. J Neurosci. 2019. PMID: 31097620 Free PMC article.
-
Deflection of a vibrissa leads to a gradient of strain across mechanoreceptors in a mystacial follicle.J Neurophysiol. 2015 Jul;114(1):138-45. doi: 10.1152/jn.00179.2015. Epub 2015 Apr 8. J Neurophysiol. 2015. PMID: 25855692 Free PMC article.
-
Constraints on the deformation of the vibrissa within the follicle.PLoS Comput Biol. 2021 Apr 1;17(4):e1007887. doi: 10.1371/journal.pcbi.1007887. eCollection 2021 Apr. PLoS Comput Biol. 2021. PMID: 33793548 Free PMC article.
-
Molecular Mechanisms of the Sense of Touch: An Overview of Mechanical Transduction and Transmission in Merkel Discs of Whisker Hair Follicles and Some Clinical Perspectives.Adv Exp Med Biol. 2018;1099:1-12. doi: 10.1007/978-981-13-1756-9_1. Adv Exp Med Biol. 2018. PMID: 30306510 Review.
-
Algorithms of whisker-mediated touch perception.Curr Opin Neurobiol. 2014 Apr;25:176-86. doi: 10.1016/j.conb.2014.01.014. Epub 2014 Feb 16. Curr Opin Neurobiol. 2014. PMID: 24549178 Review.
Cited by
-
Demonstration of three-dimensional contact point determination and contour reconstruction during active whisking behavior of an awake rat.PLoS Comput Biol. 2022 Sep 15;18(9):e1007763. doi: 10.1371/journal.pcbi.1007763. eCollection 2022 Sep. PLoS Comput Biol. 2022. PMID: 36108064 Free PMC article.
-
Sensory Adaptation in the Whisker-Mediated Tactile System: Physiology, Theory, and Function.Front Neurosci. 2021 Oct 29;15:770011. doi: 10.3389/fnins.2021.770011. eCollection 2021. Front Neurosci. 2021. PMID: 34776857 Free PMC article. Review.
-
Supra-orbital whiskers act as wind-sensing antennae in rats.PLoS Biol. 2023 Jul 6;21(7):e3002168. doi: 10.1371/journal.pbio.3002168. eCollection 2023 Jul. PLoS Biol. 2023. PMID: 37410722 Free PMC article.
-
A Vibrissa-Inspired Highly Flexible Tactile Sensor: Scanning 3D Object Surfaces Providing Tactile Images.Sensors (Basel). 2021 Feb 24;21(5):1572. doi: 10.3390/s21051572. Sensors (Basel). 2021. PMID: 33668168 Free PMC article.
-
On the intrinsic curvature of animal whiskers.PLoS One. 2023 Jan 6;18(1):e0269210. doi: 10.1371/journal.pone.0269210. eCollection 2023. PLoS One. 2023. PMID: 36607960 Free PMC article.
References
Publication types
MeSH terms
Grants and funding
LinkOut - more resources
Full Text Sources