Comparison of computational methods for the identification of topologically associating domains
- PMID: 30526631
- PMCID: PMC6288901
- DOI: 10.1186/s13059-018-1596-9
Comparison of computational methods for the identification of topologically associating domains
Abstract
Background: Chromatin folding gives rise to structural elements among which are clusters of densely interacting DNA regions termed topologically associating domains (TADs). TADs have been characterized across multiple species, tissue types, and differentiation stages, sometimes in association with regulation of biological functions. The reliability and reproducibility of these findings are intrinsically related with the correct identification of these domains from high-throughput chromatin conformation capture (Hi-C) experiments.
Results: Here, we test and compare 22 computational methods to identify TADs across 20 different conditions. We find that TAD sizes and numbers vary significantly among callers and data resolutions, challenging the definition of an average TAD size, but strengthening the hypothesis that TADs are hierarchically organized domains, rather than disjoint structural elements. Performances of these methods differ based on data resolution and normalization strategy, but a core set of TAD callers consistently retrieve reproducible domains, even at low sequencing depths, that are enriched for TAD-associated biological features.
Conclusions: This study provides a reference for the analysis of chromatin domains from Hi-C experiments and useful guidelines for choosing a suitable approach based on the experimental design, available data, and biological question of interest.
Keywords: Hi-C; Method comparison; Topologically associating domain.
Conflict of interest statement
Ethics approval and consent to participate
Not applicable.
Consent for publication
Not applicable.
Competing interests
The authors declare that they have no competing interests.
Publisher’s Note
Springer Nature remains neutral with regard to jurisdictional claims in published maps and institutional affiliations.
Figures
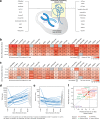
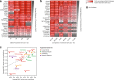
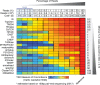
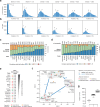
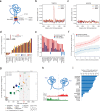
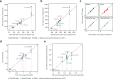
Similar articles
-
A comparison of topologically associating domain callers over mammals at high resolution.BMC Bioinformatics. 2022 Apr 12;23(1):127. doi: 10.1186/s12859-022-04674-2. BMC Bioinformatics. 2022. PMID: 35413815 Free PMC article.
-
A Comparison of Topologically Associating Domain Callers Based on Hi-C Data.IEEE/ACM Trans Comput Biol Bioinform. 2023 Jan-Feb;20(1):15-29. doi: 10.1109/TCBB.2022.3147805. Epub 2023 Feb 3. IEEE/ACM Trans Comput Biol Bioinform. 2023. PMID: 35104223
-
Methods for the Analysis of Topologically Associating Domains (TADs).Methods Mol Biol. 2022;2301:39-59. doi: 10.1007/978-1-0716-1390-0_3. Methods Mol Biol. 2022. PMID: 34415530
-
Principles of genome folding into topologically associating domains.Sci Adv. 2019 Apr 10;5(4):eaaw1668. doi: 10.1126/sciadv.aaw1668. eCollection 2019 Apr. Sci Adv. 2019. PMID: 30989119 Free PMC article. Review.
-
A TAD Skeptic: Is 3D Genome Topology Conserved?Trends Genet. 2021 Mar;37(3):216-223. doi: 10.1016/j.tig.2020.10.009. Epub 2020 Nov 14. Trends Genet. 2021. PMID: 33203573 Free PMC article. Review.
Cited by
-
HPTAD: A computational method to identify topologically associating domains from HiChIP and PLAC-seq datasets.Comput Struct Biotechnol J. 2023 Jan 9;21:931-939. doi: 10.1016/j.csbj.2023.01.003. eCollection 2023. Comput Struct Biotechnol J. 2023. PMID: 38213897 Free PMC article.
-
Mouse genomic and cellular annotations.Mamm Genome. 2022 Mar;33(1):19-30. doi: 10.1007/s00335-021-09936-7. Epub 2022 Feb 5. Mamm Genome. 2022. PMID: 35124726 Free PMC article. Review.
-
Comprehensive characterization of the embryonic factor LEUTX.iScience. 2023 Feb 9;26(3):106172. doi: 10.1016/j.isci.2023.106172. eCollection 2023 Mar 17. iScience. 2023. PMID: 36876139 Free PMC article.
-
Mapping robust multiscale communities in chromosome contact networks.Sci Rep. 2023 Aug 10;13(1):12979. doi: 10.1038/s41598-023-39522-7. Sci Rep. 2023. PMID: 37563218 Free PMC article.
-
Comparing chromatin contact maps at scale: methods and insights.Res Sq [Preprint]. 2023 May 23:rs.3.rs-2842981. doi: 10.21203/rs.3.rs-2842981/v1. Res Sq. 2023. PMID: 37292728 Free PMC article. Preprint.
References
-
- Horta A, Monahan K, Bashkirova L, Lomvardas S. Cell type-specific interchromosomal interactions as a mechanism for transcriptional diversity, bioRxiv. 10.1101/287532.
Publication types
MeSH terms
LinkOut - more resources
Full Text Sources
Other Literature Sources