Does the evolution of self-fertilization rescue populations or increase the risk of extinction?
- PMID: 30052766
- PMCID: PMC6344216
- DOI: 10.1093/aob/mcy144
Does the evolution of self-fertilization rescue populations or increase the risk of extinction?
Abstract
Background and aims: As a major evolutionary transition in seed plants, the evolution of plant mating systems has been much debated in evolutionary ecology. Over the last 10 years, well-established patterns of evolution have emerged. On the one hand, experimental studies have shown that self-fertilization is likely to evolve in a few generations (microevolution) as a response to rapid environmental change (e.g. pollinator decline), eventually rescuing a population. On the other, phylogenetic studies have demonstrated that repeated evolution towards self-fertilization (macroevolution) leads to a higher risk of lineage extinction and is thus likely to be disadvantageous in the long term.
Scope: In either case - the short-term or long-term evolution of self-fertilization (selfing) - these findings indicate that a mating system is not neutral with respect to population or lineage persistence. They also suggest that selfing can have contrasting effects depending on time scale. This raises the question of whether mating system evolution can rescue populations facing environmental change. In this review, empirical and theoretical evidence of the direct and indirect effects of mating systems on population demography and lineage persistence were analysed. A simple theoretical evolutionary rescue model was also developed to investigate the potential for evolutionary rescue through selfing.
Key findings: Demographic studies consistently show a short-term advantage of selfing provided by reproductive assurance, but a long-term disadvantage for selfing lineages, suggesting indirect genomic consequences of selfing (e.g. mutation load and lower adaptability). However, our theoretical evolutionary rescue model found that even in the short term, while mating system evolution can lead to evolutionary rescue, it can also lead to evolutionary suicide, due to the inherent frequency-dependent selection of mating system traits.
Conclusions: These findings point to the importance of analysing the demographic consequences of self-fertilization in order to predict the effect of selfing on population persistence as well as take into account the indirect genomic consequences of selfing. The pace at which processes such as inbreeding depression, purging, reproductive assurance and genomic rearrangements occur after the selfing transition is the key to clarifying whether or not selfing will result in evolutionary rescue.
Figures
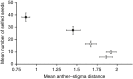
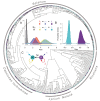
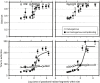
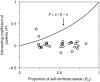
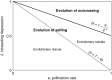
Similar articles
-
Inbreeding depression under mixed outcrossing, self-fertilization and sib-mating.BMC Evol Biol. 2016 May 17;16:105. doi: 10.1186/s12862-016-0668-2. BMC Evol Biol. 2016. PMID: 27188583 Free PMC article.
-
The double edged sword: The demographic consequences of the evolution of self-fertilization.Evolution. 2017 May;71(5):1178-1190. doi: 10.1111/evo.13222. Epub 2017 Mar 31. Evolution. 2017. PMID: 28262926
-
The relative importance of reproductive assurance and automatic selection as hypotheses for the evolution of self-fertilization.Ann Bot. 2012 Feb;109(3):553-62. doi: 10.1093/aob/mcr219. Epub 2011 Sep 20. Ann Bot. 2012. PMID: 21937484 Free PMC article.
-
Evolutionary consequences of self-fertilization in plants.Proc Biol Sci. 2013 Jun 7;280(1760):20130133. doi: 10.1098/rspb.2013.0133. Print 2013 Jun 7. Proc Biol Sci. 2013. PMID: 23595268 Free PMC article. Review.
-
The demography and population genomics of evolutionary transitions to self-fertilization in plants.Philos Trans R Soc Lond B Biol Sci. 2014 Aug 5;369(1648):20130344. doi: 10.1098/rstb.2013.0344. Philos Trans R Soc Lond B Biol Sci. 2014. PMID: 24958918 Free PMC article. Review.
Cited by
-
Not just flowering time: a resurrection approach shows floral attraction traits are changing over time.Evol Lett. 2023 Mar 8;7(2):88-98. doi: 10.1093/evlett/qrad006. eCollection 2023 Apr 1. Evol Lett. 2023. PMID: 37033876 Free PMC article.
-
Pollinator loss causes rapid adaptive evolution of selfing and dramatically reduces genome-wide genetic variability.Evolution. 2022 Sep;76(9):2130-2144. doi: 10.1111/evo.14572. Epub 2022 Jul 25. Evolution. 2022. PMID: 35852008 Free PMC article.
-
Evolution of selfing syndrome and its influence on genetic diversity and inbreeding: A range-wide study in Oenothera primiveris.Am J Bot. 2022 May;109(5):789-805. doi: 10.1002/ajb2.1861. Epub 2022 May 21. Am J Bot. 2022. PMID: 35596689 Free PMC article.
-
How rapidly do self-compatible populations evolve selfing? Mating system estimation within recently evolved self-compatible populations of Azorean Tolpis succulenta (Asteraceae).Ecol Evol. 2020 Nov 20;10(24):13990-13999. doi: 10.1002/ece3.6992. eCollection 2020 Dec. Ecol Evol. 2020. PMID: 33391697 Free PMC article.
-
Spatial Patterns and Drivers of Angiosperm Sexual Systems in China Differ Between Woody and Herbaceous Species.Front Plant Sci. 2020 Aug 11;11:1222. doi: 10.3389/fpls.2020.01222. eCollection 2020. Front Plant Sci. 2020. PMID: 32849756 Free PMC article.
References
-
- Abu Awad D, Billiard S. 2017. The double edged sword: the demographic consequences of the evolution of self-fertilization. Evolution 71: 1178–1190. - PubMed
-
- Aguilar R, Ashworth L, Galetto L, Aizen MA. 2006. Plant reproductive susceptibility to habitat fragmentation: review and synthesis through a meta-analysis. Ecology Letters 9: 968–980. - PubMed
-
- Armbruster WS. 1988. Multilevel comparative-analysis of the morphology, function, and evolution of Dalechampia blossoms. Ecology 69: 1746–1761.
-
- Ashman TL, Majetic CJ. 2006. Genetic constraints on floral evolution: a review and evaluation of patterns. Heredity 96: 343–352. - PubMed
-
- Baker HG. 1955. Self-compatibility and establishment after ‘long distance’ dispersal. Evolution 9: 347–348.
Publication types
MeSH terms
LinkOut - more resources
Full Text Sources
Other Literature Sources
Research Materials