Genomewide binding of transcription factor Snail1 in triple-negative breast cancer cells
- PMID: 29729076
- PMCID: PMC6026864
- DOI: 10.1002/1878-0261.12317
Genomewide binding of transcription factor Snail1 in triple-negative breast cancer cells
Abstract
Transcriptional regulation mediated by the zinc finger protein Snail1 controls early embryogenesis. By binding to the epithelial tumor suppressor CDH1 gene, Snail1 initiates the epithelial-mesenchymal transition (EMT). The EMT generates stem-like cells and promotes invasiveness during cancer progression. Accordingly, Snail1 mRNA and protein is abundantly expressed in triple-negative breast cancers with enhanced metastatic potential and phenotypic signs of the EMT. Such high endogenous Snail1 protein levels permit quantitative chromatin immunoprecipitation-sequencing (ChIP-seq) analysis. Snail1 associated with 185 genes at cis regulatory regions in the Hs578T triple-negative breast cancer cell model. These genes include morphogenetic regulators and signaling components that control polarized differentiation. Using the CRISPR/Cas9 system in Hs578T cells, a double deletion of 10 bp each was engineered into the first exon and into the second exon-intron junction of Snail1, suppressing Snail1 expression and causing misregulation of several hundred genes. Specific attention to regulators of chromatin organization provides a possible link to new phenotypes uncovered by the Snail1 loss-of-function mutation. On the other hand, genetic inactivation of Snail1 was not sufficient to establish a full epithelial transition to these tumor cells. Thus, Snail1 contributes to the malignant phenotype of breast cancer cells via diverse new mechanisms.
Keywords: bone morphogenetic protein; breast cancer; chromatin immunoprecipitation; epithelial-mesenchymal transition; transforming growth factor β.
© 2018 The Authors. Published by FEBS Press and John Wiley & Sons Ltd.
Figures
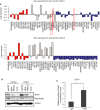
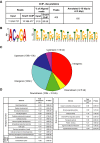
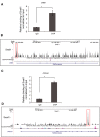
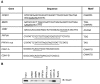
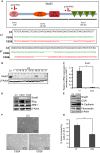
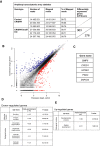
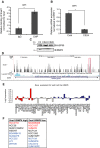
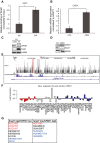
Similar articles
-
SNAIL1-mediated downregulation of FOXA proteins facilitates the inactivation of transcriptional enhancer elements at key epithelial genes in colorectal cancer cells.PLoS Genet. 2017 Nov 20;13(11):e1007109. doi: 10.1371/journal.pgen.1007109. eCollection 2017 Nov. PLoS Genet. 2017. PMID: 29155818 Free PMC article.
-
ZEB1 is neither sufficient nor required for epithelial-mesenchymal transition in LS174T colorectal cancer cells.Biochem Biophys Res Commun. 2017 Jan 22;482(4):1226-1232. doi: 10.1016/j.bbrc.2016.12.017. Epub 2016 Dec 5. Biochem Biophys Res Commun. 2017. PMID: 27923654
-
Genome-wide binding of transcription factor ZEB1 in triple-negative breast cancer cells.J Cell Physiol. 2018 Oct;233(10):7113-7127. doi: 10.1002/jcp.26634. Epub 2018 May 10. J Cell Physiol. 2018. PMID: 29744893 Free PMC article.
-
The emerging role of Snail1 in the tumor stroma.Clin Transl Oncol. 2016 Sep;18(9):872-7. doi: 10.1007/s12094-015-1474-9. Epub 2015 Dec 21. Clin Transl Oncol. 2016. PMID: 26687368 Review.
-
Central role of Snail1 in the regulation of EMT and resistance in cancer: a target for therapeutic intervention.J Exp Clin Cancer Res. 2014 Aug 2;33(1):62. doi: 10.1186/s13046-014-0062-0. J Exp Clin Cancer Res. 2014. PMID: 25084828 Free PMC article. Review.
Cited by
-
Expression and clinical significance of WWOX, Elf5, Snail1 and EMT related factors in epithelial ovarian cancer.Oncol Lett. 2020 Feb;19(2):1281-1290. doi: 10.3892/ol.2019.11213. Epub 2019 Dec 12. Oncol Lett. 2020. PMID: 31966058 Free PMC article.
-
MicroRNA-138-5p targets the NFIB-Snail1 axis to inhibit colorectal cancer cell migration and chemoresistance.Cancer Cell Int. 2020 Oct 1;20:475. doi: 10.1186/s12935-020-01573-5. eCollection 2020. Cancer Cell Int. 2020. PMID: 33013202 Free PMC article.
-
Epithelial-Mesenchymal Transition and Metastasis under the Control of Transforming Growth Factor β.Int J Mol Sci. 2018 Nov 20;19(11):3672. doi: 10.3390/ijms19113672. Int J Mol Sci. 2018. PMID: 30463358 Free PMC article. Review.
-
The Developmental Origins of Cancer: A Review of the Genes Expressed in Embryonic Cells with Implications for Tumorigenesis.Genes (Basel). 2023 Feb 28;14(3):604. doi: 10.3390/genes14030604. Genes (Basel). 2023. PMID: 36980876 Free PMC article. Review.
-
Loss of SNAI1 induces cellular plasticity in invasive triple-negative breast cancer cells.Cell Death Dis. 2022 Sep 28;13(9):832. doi: 10.1038/s41419-022-05280-z. Cell Death Dis. 2022. PMID: 36171192 Free PMC article.
References
-
- Astro V, Asperti C, Cangi MG, Doglioni C and de Curtis I (2011) Liprin‐a1 regulates breast cancer cell invasion by affecting cell motility, invadopodia and extracellular matrix degradation. Oncogene 30, 1841–1849. - PubMed
-
- Barrallo‐Gimeno A and Nieto MA (2009) Evolutionary history of the Snail/Scratch superfamily. Trends Genet 25, 248–252. - PubMed
Publication types
MeSH terms
Substances
LinkOut - more resources
Full Text Sources
Other Literature Sources
Research Materials
Miscellaneous