Active Touch and Self-Motion Encoding by Merkel Cell-Associated Afferents
- PMID: 28434802
- PMCID: PMC5528144
- DOI: 10.1016/j.neuron.2017.03.045
Active Touch and Self-Motion Encoding by Merkel Cell-Associated Afferents
Abstract
Touch perception depends on integrating signals from multiple types of peripheral mechanoreceptors. Merkel-cell associated afferents are thought to play a major role in form perception by encoding surface features of touched objects. However, activity of Merkel afferents during active touch has not been directly measured. Here, we show that Merkel and unidentified slowly adapting afferents in the whisker system of behaving mice respond to both self-motion and active touch. Touch responses were dominated by sensitivity to bending moment (torque) at the base of the whisker and its rate of change and largely explained by a simple mechanical model. Self-motion responses encoded whisker position within a whisk cycle (phase), not absolute whisker angle, and arose from stresses reflecting whisker inertia and activity of specific muscles. Thus, Merkel afferents send to the brain multiplexed information about whisker position and surface features, suggesting that proprioception and touch converge at the earliest neural level.
Keywords: active sensation; barrel cortex; neural coding; perception; primary afferents; proprioception; reafferent; sensorimotor integration; somatosensation; whisker system.
Copyright © 2017 Elsevier Inc. All rights reserved.
Figures
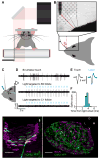
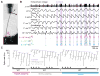
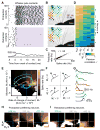
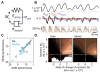
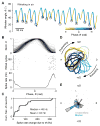
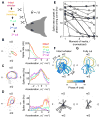
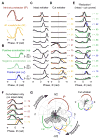
Similar articles
-
Independent representations of self-motion and object location in barrel cortex output.PLoS Biol. 2020 Nov 3;18(11):e3000882. doi: 10.1371/journal.pbio.3000882. eCollection 2020 Nov. PLoS Biol. 2020. PMID: 33141817 Free PMC article.
-
Epidermal Merkel cells are mechanosensory cells that tune mammalian touch receptors.Nature. 2014 May 29;509(7502):617-21. doi: 10.1038/nature13250. Epub 2014 Apr 6. Nature. 2014. PMID: 24717432 Free PMC article.
-
Coding of whisker motion across the mouse face.Elife. 2019 Feb 28;8:e41535. doi: 10.7554/eLife.41535. Elife. 2019. PMID: 30816844 Free PMC article.
-
What the whiskers tell the brain.Neuroscience. 2018 Jan 1;368:95-108. doi: 10.1016/j.neuroscience.2017.08.005. Epub 2017 Aug 24. Neuroscience. 2018. PMID: 28843998 Review.
-
'Where' and 'what' in the whisker sensorimotor system.Nat Rev Neurosci. 2008 Aug;9(8):601-12. doi: 10.1038/nrn2411. Nat Rev Neurosci. 2008. PMID: 18641667 Review.
Cited by
-
Cortical circuitry mediating interareal touch signal amplification.Cell Rep. 2023 Dec 26;42(12):113532. doi: 10.1016/j.celrep.2023.113532. Epub 2023 Dec 6. Cell Rep. 2023. PMID: 38064338 Free PMC article.
-
Prediction of Choice from Competing Mechanosensory and Choice-Memory Cues during Active Tactile Decision Making.J Neurosci. 2019 May 15;39(20):3921-3933. doi: 10.1523/JNEUROSCI.2217-18.2019. Epub 2019 Mar 8. J Neurosci. 2019. PMID: 30850514 Free PMC article.
-
Whisker Vibrations and the Activity of Trigeminal Primary Afferents in Response to Airflow.J Neurosci. 2019 Jul 24;39(30):5881-5896. doi: 10.1523/JNEUROSCI.2971-18.2019. Epub 2019 May 16. J Neurosci. 2019. PMID: 31097620 Free PMC article.
-
A machine-vision approach for automated pain measurement at millisecond timescales.Elife. 2020 Aug 6;9:e57258. doi: 10.7554/eLife.57258. Elife. 2020. PMID: 32758355 Free PMC article.
-
Interactions of Whisking and Touch Signals in the Rat Brainstem.J Neurosci. 2021 Jun 2;41(22):4826-4839. doi: 10.1523/JNEUROSCI.1410-20.2021. Epub 2021 Apr 23. J Neurosci. 2021. PMID: 33893218 Free PMC article.
References
-
- Bagdasarian K, Szwed M, Knutsen PM, Deutsch D, Derdikman D, Pietr M, Simony E, Ahissar E. Pre-neuronal morphological processing of object location by individual whiskers. Nat Neurosci. 2013;16:622–631. - PubMed
-
- Bermejo R, Szwed M, Friedman W, Ahissar E, Zeigler HP. One whisker whisking: unit recording during conditioned whisking in rats. Somatosens Mot Res. 2004;21:183–187. - PubMed
MeSH terms
Grants and funding
LinkOut - more resources
Full Text Sources
Other Literature Sources
Medical
Molecular Biology Databases
Miscellaneous