Genetic silencing of olivocerebellar synapses causes dystonia-like behaviour in mice
- PMID: 28374839
- PMCID: PMC5382291
- DOI: 10.1038/ncomms14912
Genetic silencing of olivocerebellar synapses causes dystonia-like behaviour in mice
Abstract
Theories of cerebellar function place the inferior olive to cerebellum connection at the centre of motor behaviour. One possible implication of this is that disruption of olivocerebellar signalling could play a major role in initiating motor disease. To test this, we devised a mouse genetics approach to silence glutamatergic signalling only at olivocerebellar synapses. The resulting mice had a severe neurological condition that mimicked the early-onset twisting, stiff limbs and tremor that is observed in dystonia, a debilitating movement disease. By blocking olivocerebellar excitatory neurotransmission, we eliminated Purkinje cell complex spikes and induced aberrant cerebellar nuclear activity. Pharmacologically inhibiting the erratic output of the cerebellar nuclei in the mutant mice improved movement. Furthermore, deep brain stimulation directed to the interposed cerebellar nuclei reduced dystonia-like postures in these mice. Collectively, our data uncover a neural mechanism by which olivocerebellar dysfunction promotes motor disease phenotypes and identify the cerebellar nuclei as a therapeutic target for surgical intervention.
Conflict of interest statement
The authors declare no competing financial interests.
Figures
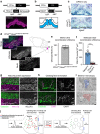
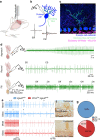
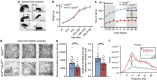
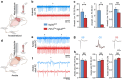
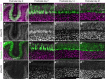
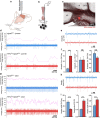
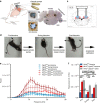
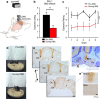
Similar articles
-
Cerebellar modules in the olivo-cortico-nuclear loop demarcated by pcdh10 expression in the adult mouse.J Comp Neurol. 2018 Oct 15;526(15):2406-2427. doi: 10.1002/cne.24499. Epub 2018 Oct 8. J Comp Neurol. 2018. PMID: 30004589
-
Subtype switching of vesicular glutamate transporters at parallel fibre-Purkinje cell synapses in developing mouse cerebellum.Eur J Neurosci. 2003 Jun;17(12):2563-72. doi: 10.1046/j.1460-9568.2003.02698.x. Eur J Neurosci. 2003. PMID: 12823463
-
Deep Brain Stimulation of the Interposed Cerebellar Nuclei in a Conditional Genetic Mouse Model with Dystonia.Adv Neurobiol. 2023;31:93-117. doi: 10.1007/978-3-031-26220-3_6. Adv Neurobiol. 2023. PMID: 37338698
-
Excitatory afferent modulation of complex spike synchrony.Cerebellum. 2003;2(3):165-70. doi: 10.1080/14734220310002542. Cerebellum. 2003. PMID: 14509565 Review.
-
[Mechanisms of modification of excitatory and inhibitory inputs in various neurons of olivary-cerebellar network].Zh Vyssh Nerv Deiat Im I P Pavlova. 2000 May-Jun;50(3):372-87. Zh Vyssh Nerv Deiat Im I P Pavlova. 2000. PMID: 10923375 Review. Russian.
Cited by
-
Abnormal Cerebellar Development in Autism Spectrum Disorders.Dev Neurosci. 2021;43(3-4):181-190. doi: 10.1159/000515189. Epub 2021 Apr 6. Dev Neurosci. 2021. PMID: 33823515 Free PMC article. Review.
-
Acute cerebellar knockdown of Sgce reproduces salient features of myoclonus-dystonia (DYT11) in mice.Elife. 2019 Dec 23;8:e52101. doi: 10.7554/eLife.52101. Elife. 2019. PMID: 31868164 Free PMC article.
-
Molecular layer interneurons shape the spike activity of cerebellar Purkinje cells.Sci Rep. 2019 Feb 11;9(1):1742. doi: 10.1038/s41598-018-38264-1. Sci Rep. 2019. PMID: 30742002 Free PMC article.
-
ATXN1-CIC Complex Is the Primary Driver of Cerebellar Pathology in Spinocerebellar Ataxia Type 1 through a Gain-of-Function Mechanism.Neuron. 2018 Mar 21;97(6):1235-1243.e5. doi: 10.1016/j.neuron.2018.02.013. Epub 2018 Mar 8. Neuron. 2018. PMID: 29526553 Free PMC article.
-
Computational models of neurotransmission at cerebellar synapses unveil the impact on network computation.Front Comput Neurosci. 2022 Oct 28;16:1006989. doi: 10.3389/fncom.2022.1006989. eCollection 2022. Front Comput Neurosci. 2022. PMID: 36387305 Free PMC article. Review.
References
-
- Breakefield X. O. et al.. The pathophysiological basis of dystonias. Nat. Rev. Neurosci. 9, 222–234 (2008). - PubMed
Publication types
MeSH terms
Substances
Grants and funding
LinkOut - more resources
Full Text Sources
Other Literature Sources
Medical
Molecular Biology Databases