BRD4 mediates NF-κB-dependent epithelial-mesenchymal transition and pulmonary fibrosis via transcriptional elongation
- PMID: 27793799
- PMCID: PMC5206405
- DOI: 10.1152/ajplung.00224.2016
BRD4 mediates NF-κB-dependent epithelial-mesenchymal transition and pulmonary fibrosis via transcriptional elongation
Abstract
Chronic epithelial injury triggers a TGF-β-mediated cellular transition from normal epithelium into a mesenchymal-like state that produces subepithelial fibrosis and airway remodeling. Here we examined how TGF-β induces the mesenchymal cell state and determined its mechanism. We observed that TGF-β stimulation activates an inflammatory gene program controlled by the NF-κB/RelA signaling pathway. In the mesenchymal state, NF-κB-dependent immediate-early genes accumulate euchromatin marks and processive RNA polymerase. This program of immediate-early genes is activated by enhanced expression, nuclear translocation, and activating phosphorylation of the NF-κB/RelA transcription factor on Ser276, mediated by a paracrine signal. Phospho-Ser276 RelA binds to the BRD4/CDK9 transcriptional elongation complex, activating the paused RNA Pol II by phosphorylation on Ser2 in its carboxy-terminal domain. RelA-initiated transcriptional elongation is required for expression of the core epithelial-mesenchymal transition transcriptional regulators SNAI1, TWIST1, and ZEB1 and mesenchymal genes. Finally, we observed that pharmacological inhibition of BRD4 can attenuate experimental lung fibrosis induced by repetitive TGF-β challenge in a mouse model. These data provide a detailed mechanism for how activated NF-κB and BRD4 control epithelial-mesenchymal transition initiation and transcriptional elongation in model airway epithelial cells in vitro and in a murine pulmonary fibrosis model in vivo. Our data validate BRD4 as an in vivo target for the treatment of pulmonary fibrosis associated with inflammation-coupled remodeling in chronic lung diseases.
Keywords: BRD4; airway epithelial cells; fibrosis; mesenchymal transition; nuclear factor-κB.
Copyright © 2016 the American Physiological Society.
Figures
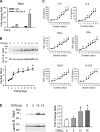
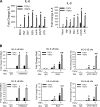
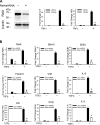
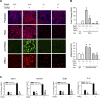
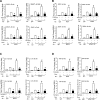
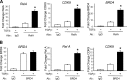
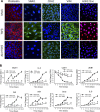
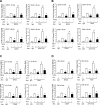
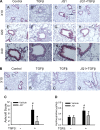
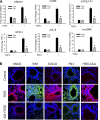
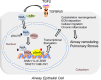
Similar articles
-
BRD4 Couples NF-κB/RelA with Airway Inflammation and the IRF-RIG-I Amplification Loop in Respiratory Syncytial Virus Infection.J Virol. 2017 Feb 28;91(6):e00007-17. doi: 10.1128/JVI.00007-17. Print 2017 Mar 15. J Virol. 2017. PMID: 28077651 Free PMC article.
-
Efficacy of Novel Highly Specific Bromodomain-Containing Protein 4 Inhibitors in Innate Inflammation-Driven Airway Remodeling.Am J Respir Cell Mol Biol. 2019 Jan;60(1):68-83. doi: 10.1165/rcmb.2017-0445OC. Am J Respir Cell Mol Biol. 2019. PMID: 30153047 Free PMC article.
-
NF-κB Mediates Mesenchymal Transition, Remodeling, and Pulmonary Fibrosis in Response to Chronic Inflammation by Viral RNA Patterns.Am J Respir Cell Mol Biol. 2017 Apr;56(4):506-520. doi: 10.1165/rcmb.2016-0259OC. Am J Respir Cell Mol Biol. 2017. PMID: 27911568 Free PMC article.
-
Targeting Chromatin Remodeling in Inflammation and Fibrosis.Adv Protein Chem Struct Biol. 2017;107:1-36. doi: 10.1016/bs.apcsb.2016.11.001. Epub 2016 Dec 1. Adv Protein Chem Struct Biol. 2017. PMID: 28215221 Review.
-
Research Advances in the Role of Bromodomain-containing Protein 4 in Epithelial-mesenchymal Transition in Asthma.Zhongguo Yi Xue Ke Xue Yuan Xue Bao. 2017 Jun 20;39(3):425-431. doi: 10.3881/j.issn.1000-503X.2017.03.022. Zhongguo Yi Xue Ke Xue Yuan Xue Bao. 2017. PMID: 28695816 Review.
Cited by
-
Genetic or pharmacologic blockade of enhancer of zeste homolog 2 inhibits the progression of peritoneal fibrosis.J Pathol. 2020 Jan;250(1):79-94. doi: 10.1002/path.5352. Epub 2019 Nov 14. J Pathol. 2020. PMID: 31579944 Free PMC article.
-
Therapeutic targets for inflammation-mediated airway remodeling in chronic lung disease.Expert Rev Respir Med. 2018 Nov;12(11):931-939. doi: 10.1080/17476348.2018.1526677. Epub 2018 Oct 3. Expert Rev Respir Med. 2018. PMID: 30241450 Free PMC article. Review.
-
Role of Secretoglobin+ (club cell) NFκB/RelA-TGFβ signaling in aero-allergen-induced epithelial plasticity and subepithelial myofibroblast transdifferentiation.Respir Res. 2021 Dec 20;22(1):315. doi: 10.1186/s12931-021-01910-w. Respir Res. 2021. PMID: 34930252 Free PMC article.
-
Bromodomain Containing Protein 4 (BRD4) Regulates Expression of its Interacting Coactivators in the Innate Response to Respiratory Syncytial Virus.Front Mol Biosci. 2021 Oct 26;8:728661. doi: 10.3389/fmolb.2021.728661. eCollection 2021. Front Mol Biosci. 2021. PMID: 34765643 Free PMC article.
-
Astragalus polysaccharides attenuate pulmonary fibrosis by inhibiting the epithelial-mesenchymal transition and NF-κB pathway activation.Int J Mol Med. 2020 Jul;46(1):331-339. doi: 10.3892/ijmm.2020.4574. Epub 2020 Apr 13. Int J Mol Med. 2020. PMID: 32319542 Free PMC article.
References
-
- Al-Muhsen S, Johnson JR, Hamid Q. Remodeling in asthma. J Allergy Clin Immunol 128: 451–462, 2011. - PubMed
-
- Alexopoulou L, Holt AC, Medzhitov R, Flavell RA. Recognition of double-stranded RNA and activation of NF-kappaB by Toll-like receptor 3. Nature 413: 732–738, 2001. - PubMed
-
- Bousquet J, Jeffery PK, Busse WW, Johnson M, Vignola AM. Asthma. From bronchoconstriction to airways inflammation and remodeling. Am J Respir Crit Care Med 161: 1720–1745, 2000. - PubMed
MeSH terms
Substances
Grants and funding
LinkOut - more resources
Full Text Sources
Other Literature Sources
Medical
Research Materials
Miscellaneous