Multi-decadal trends in global terrestrial evapotranspiration and its components
- PMID: 26750505
- PMCID: PMC4707530
- DOI: 10.1038/srep19124
Multi-decadal trends in global terrestrial evapotranspiration and its components
Abstract
Evapotranspiration (ET) is the process by which liquid water becomes water vapor and energetically this accounts for much of incoming solar radiation. If this ET did not occur temperatures would be higher, so understanding ET trends is crucial to predict future temperatures. Recent studies have reported prolonged declines in ET in recent decades, although these declines may relate to climate variability. Here, we used a well-validated diagnostic model to estimate daily ET during 1981-2012, and its three components: transpiration from vegetation (Et), direct evaporation from the soil (Es) and vaporization of intercepted rainfall from vegetation (Ei). During this period, ET over land has increased significantly (p < 0.01), caused by increases in Et and Ei, which are partially counteracted by Es decreasing. These contrasting trends are primarily driven by increases in vegetation leaf area index, dominated by greening. The overall increase in Et over land is about twofold of the decrease in Es. These opposing trends are not simulated by most Coupled Model Intercomparison Project phase 5 (CMIP5) models, and highlight the importance of realistically representing vegetation changes in earth system models for predicting future changes in the energy and water cycle.
Figures
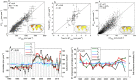
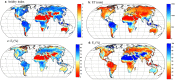
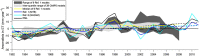
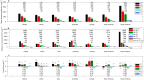
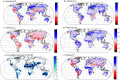
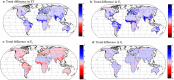
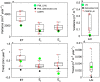
Similar articles
-
Discriminating the impacts of vegetation greening and climate change on the changes in evapotranspiration and transpiration fraction over the Yellow River Basin.Sci Total Environ. 2023 Dec 15;904:166926. doi: 10.1016/j.scitotenv.2023.166926. Epub 2023 Sep 7. Sci Total Environ. 2023. PMID: 37689185
-
The role of climate change and vegetation greening on the variation of terrestrial evapotranspiration in northwest China's Qilian Mountains.Sci Total Environ. 2021 Mar 10;759:143532. doi: 10.1016/j.scitotenv.2020.143532. Epub 2020 Nov 16. Sci Total Environ. 2021. PMID: 33250260
-
Vegetation Greening and Climate Change Promote Multidecadal Rises of Global Land Evapotranspiration.Sci Rep. 2015 Oct 30;5:15956. doi: 10.1038/srep15956. Sci Rep. 2015. PMID: 26514110 Free PMC article.
-
Estimating evapotranspiration and drought stress with ground-based thermal remote sensing in agriculture: a review.J Exp Bot. 2012 Aug;63(13):4671-712. doi: 10.1093/jxb/ers165. J Exp Bot. 2012. PMID: 22922637 Review.
-
The influence of terrestrial ecosystems on climate.Trends Ecol Evol. 2006 May;21(5):254-60. doi: 10.1016/j.tree.2006.03.005. Epub 2006 Mar 29. Trends Ecol Evol. 2006. PMID: 16697911 Review.
Cited by
-
Comprehensive evaluation of nine evapotranspiration products from remote sensing, gauge upscaling and land surface model over China.PLoS One. 2024 Nov 13;19(11):e0313762. doi: 10.1371/journal.pone.0313762. eCollection 2024. PLoS One. 2024. PMID: 39536035 Free PMC article.
-
Water limitation drives species loss in grassland communities after nitrogen addition and warming.Proc Biol Sci. 2024 Sep;291(2031):20240642. doi: 10.1098/rspb.2024.0642. Epub 2024 Sep 18. Proc Biol Sci. 2024. PMID: 39288804
-
Future climate doubles the risk of hydraulic failure in a wet tropical forest.New Phytol. 2024 Dec;244(6):2239-2250. doi: 10.1111/nph.19956. Epub 2024 Jul 18. New Phytol. 2024. PMID: 39030765 Free PMC article.
-
Groundwater-dependent ecosystem map exposes global dryland protection needs.Nature. 2024 Aug;632(8023):101-107. doi: 10.1038/s41586-024-07702-8. Epub 2024 Jul 17. Nature. 2024. PMID: 39020182 Free PMC article.
-
A global meta-analysis on the effects of organic and inorganic fertilization on grasslands and croplands.Nat Commun. 2024 Apr 22;15(1):3411. doi: 10.1038/s41467-024-47829-w. Nat Commun. 2024. PMID: 38649721 Free PMC article.
References
-
- Trenberth K. E., Smith L., Qian T. T., Dai A. & Fasullo J. Estimates of the global water budget and its annual cycle using observational and model data. Journal of Hydrometeorology 8, 758–769, doi: 10.1175/jhm600.1 (2007). - DOI
-
- Wang K. & Dickinson R. E. A review of global terrestrial evapotranspiration: Observation, modeling, climatology, and climatic variability. Reviews of Geophysics 50 (2012).
-
- Douville H., Ribes A., Decharme B., Alkama R. & Sheffield J. Anthropogenic influence on multidecadal changes in reconstructed global evapotranspiration. Nature Clim. Change 3, 59–62 (2013).
Publication types
LinkOut - more resources
Full Text Sources
Other Literature Sources