Deflection of a vibrissa leads to a gradient of strain across mechanoreceptors in a mystacial follicle
- PMID: 25855692
- PMCID: PMC4507969
- DOI: 10.1152/jn.00179.2015
Deflection of a vibrissa leads to a gradient of strain across mechanoreceptors in a mystacial follicle
Abstract
Rodents use their vibrissae to detect and discriminate tactile features during active exploration. The site of mechanical transduction in the vibrissa sensorimotor system is the follicle sinus complex and its associated vibrissa. We study the mechanics within the ring sinus (RS) of the follicle in an ex vivo preparation of the mouse mystacial pad. The sinus region has a relatively dense representation of Merkel mechanoreceptors and longitudinal lanceolate endings. Two-photon laser-scanning microscopy was used to visualize labeled cell nuclei in an ∼ 100-nl vol before and after passive deflection of a vibrissa, which results in localized displacements of the mechanoreceptor cells, primarily in the radial and polar directions about the vibrissa. These displacements are used to compute the strain field across the follicle in response to the deflection. We observe compression in the lower region of the RS, whereas dilation, with lower magnitude, occurs in the upper region, with volumetric strain ΔV/V ∼ 0.01 for a 10° deflection. The extrapolated strain for a 0.1° deflection, the minimum angle that is reported to initiate a spike by primary neurons, corresponds to the minimum strain that activates Piezo2 mechanoreceptor channels.
Keywords: Merkel cells; biomechanics; displacement; ringwulst; somatosensation; whisker.
Copyright © 2015 the American Physiological Society.
Figures
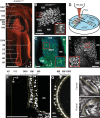
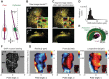
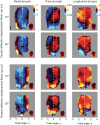
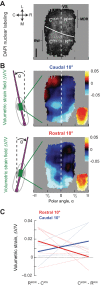
Similar articles
-
Constraints on the deformation of the vibrissa within the follicle.PLoS Comput Biol. 2021 Apr 1;17(4):e1007887. doi: 10.1371/journal.pcbi.1007887. eCollection 2021 Apr. PLoS Comput Biol. 2021. PMID: 33793548 Free PMC article.
-
Similarities and differences in the innervation of mystacial vibrissal follicle-sinus complexes in the rat and cat: a confocal microscopic study.J Comp Neurol. 2002 Jul 22;449(2):103-19. doi: 10.1002/cne.10277. J Comp Neurol. 2002. PMID: 12115682
-
Adaptations in the structure and innervation of follicle-sinus complexes to an aquatic environment as seen in the Florida manatee (Trichechus manatus latirostris).J Comp Neurol. 2007 Sep 20;504(3):217-37. doi: 10.1002/cne.21446. J Comp Neurol. 2007. PMID: 17640045
-
Molecular Mechanisms of the Sense of Touch: An Overview of Mechanical Transduction and Transmission in Merkel Discs of Whisker Hair Follicles and Some Clinical Perspectives.Adv Exp Med Biol. 2018;1099:1-12. doi: 10.1007/978-981-13-1756-9_1. Adv Exp Med Biol. 2018. PMID: 30306510 Review.
-
Diversification and specialization of touch receptors in skin.Cold Spring Harb Perspect Med. 2014 Jun 2;4(6):a013656. doi: 10.1101/cshperspect.a013656. Cold Spring Harb Perspect Med. 2014. PMID: 24890830 Free PMC article. Review.
Cited by
-
Reducing Merkel cell activity in the whisker follicle disrupts cortical encoding of whisker movement amplitude and velocity.IBRO Neurosci Rep. 2022 Sep 29;13:356-363. doi: 10.1016/j.ibneur.2022.09.008. eCollection 2022 Dec. IBRO Neurosci Rep. 2022. PMID: 36281438 Free PMC article.
-
Constraints on the deformation of the vibrissa within the follicle.PLoS Comput Biol. 2021 Apr 1;17(4):e1007887. doi: 10.1371/journal.pcbi.1007887. eCollection 2021 Apr. PLoS Comput Biol. 2021. PMID: 33793548 Free PMC article.
-
Continuous, multidimensional coding of 3D complex tactile stimuli by primary sensory neurons of the vibrissal system.Proc Natl Acad Sci U S A. 2021 Aug 10;118(32):e2020194118. doi: 10.1073/pnas.2020194118. Proc Natl Acad Sci U S A. 2021. PMID: 34353902 Free PMC article.
-
Behavioral and Neural Bases of Tactile Shape Discrimination Learning in Head-Fixed Mice.Neuron. 2020 Dec 9;108(5):953-967.e8. doi: 10.1016/j.neuron.2020.09.012. Epub 2020 Sep 30. Neuron. 2020. PMID: 33002411 Free PMC article.
-
Whisking Kinematics Enables Object Localization in Head-Centered Coordinates Based on Tactile Information from a Single Vibrissa.Front Behav Neurosci. 2016 Jul 19;10:145. doi: 10.3389/fnbeh.2016.00145. eCollection 2016. Front Behav Neurosci. 2016. PMID: 27486390 Free PMC article.
References
-
- Bagdasarian K, Szwed M, Knutsen PM, Deutsch D, Derdikman D, Pietr M, Simony E, Ahissar E. Pre-neuronal morphological processing of object location by individual whiskers. Nat Neurosci 16: 622–631, 2013. - PubMed
-
- Ebara S, Kumamoto K, Matsuura T, Mazurkiewicz JE, Rice FL. Similarities and differences in the innervation of mystacial vibrissal follicle-sinus complexes in the rat and cat: a confocal microscopic study. J Comp Neurol 449: 103–119, 2002. - PubMed
Publication types
MeSH terms
Grants and funding
LinkOut - more resources
Full Text Sources
Other Literature Sources