Organ-on-a-chip platforms for studying drug delivery systems
- PMID: 24818770
- PMCID: PMC4142092
- DOI: 10.1016/j.jconrel.2014.05.004
Organ-on-a-chip platforms for studying drug delivery systems
Abstract
Novel microfluidic tools allow new ways to manufacture and test drug delivery systems. Organ-on-a-chip systems - microscale recapitulations of complex organ functions - promise to improve the drug development pipeline. This review highlights the importance of integrating microfluidic networks with 3D tissue engineered models to create organ-on-a-chip platforms, able to meet the demand of creating robust preclinical screening models. Specific examples are cited to demonstrate the use of these systems for studying the performance of drug delivery vectors and thereby reduce the discrepancies between their performance at preclinical and clinical trials. We also highlight the future directions that need to be pursued by the research community for these proof-of-concept studies to achieve the goal of accelerating clinical translation of drug delivery nanoparticles.
Keywords: Drug delivery; Drug screening; Nanoparticle; Organ-on-a-chip; Toxicity.
Copyright © 2014 Elsevier B.V. All rights reserved.
Figures
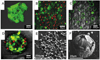
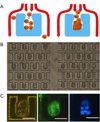
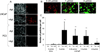
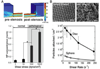
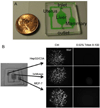
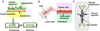
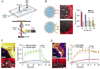
Similar articles
-
Microfluidics in nanoparticle drug delivery; From synthesis to pre-clinical screening.Adv Drug Deliv Rev. 2018 Mar 15;128:29-53. doi: 10.1016/j.addr.2018.04.001. Epub 2018 Apr 5. Adv Drug Deliv Rev. 2018. PMID: 29626551 Review.
-
Microengineered Organ-on-a-chip Platforms towards Personalized Medicine.Curr Pharm Des. 2018;24(45):5354-5366. doi: 10.2174/1381612825666190222143542. Curr Pharm Des. 2018. PMID: 30799783 Review.
-
Microfluidic Organ-on-a-Chip Technology for Advancement of Drug Development and Toxicology.Adv Healthc Mater. 2015 Jul 15;4(10):1426-50. doi: 10.1002/adhm.201500040. Epub 2015 Mar 26. Adv Healthc Mater. 2015. PMID: 25820344 Review.
-
Organ-on-a-Chip: A Preclinical Microfluidic Platform for the Progress of Nanomedicine.Small. 2020 Dec;16(51):e2003517. doi: 10.1002/smll.202003517. Epub 2020 Nov 25. Small. 2020. PMID: 33236819 Review.
-
Organ-on-a-Chip Systems: Microengineering to Biomimic Living Systems.Small. 2016 May;12(17):2253-82. doi: 10.1002/smll.201503208. Epub 2016 Feb 22. Small. 2016. PMID: 26901595 Review.
Cited by
-
Recent Advances in Micro-Electro-Mechanical Devices for Controlled Drug Release Applications.Front Bioeng Biotechnol. 2020 Jul 29;8:827. doi: 10.3389/fbioe.2020.00827. eCollection 2020. Front Bioeng Biotechnol. 2020. PMID: 32850709 Free PMC article. Review.
-
Proliferation characteristics of cells cultured under periodic versus static conditions.Cytotechnology. 2019 Feb;71(1):443-452. doi: 10.1007/s10616-018-0263-z. Epub 2018 Dec 4. Cytotechnology. 2019. PMID: 30515656 Free PMC article.
-
Metastasis-on-a-chip mimicking the progression of kidney cancer in the liver for predicting treatment efficacy.Theranostics. 2020 Jan 1;10(1):300-311. doi: 10.7150/thno.38736. eCollection 2020. Theranostics. 2020. PMID: 31903121 Free PMC article.
-
Engineering Theranostic Microbubbles Using Microfluidics for Ultrasound Imaging and Therapy: A Review.Ultrasound Med Biol. 2018 Dec;44(12):2441-2460. doi: 10.1016/j.ultrasmedbio.2018.07.026. Epub 2018 Sep 19. Ultrasound Med Biol. 2018. PMID: 30241729 Free PMC article. Review.
-
A Microfluidic Chip Embracing a Nanofiber Scaffold for 3D Cell Culture and Real-Time Monitoring.Nanomaterials (Basel). 2019 Apr 10;9(4):588. doi: 10.3390/nano9040588. Nanomaterials (Basel). 2019. PMID: 30974794 Free PMC article.
References
-
- Farokhzad OC, Langer R. Nanomedicine: developing smarter therapeutic and diagnostic modalities. Adv Drug Deliv Rev. 2006;58:1456–1459. - PubMed
-
- Peppas NA. Intelligent therapeutics: biomimetic systems and nanotechnology in drug delivery. Adv Drug Deliv Rev. 2004;56:1529–1531. - PubMed
-
- Gregoriadis G. Drug entrapment in liposomes. FEBS Lett. 1973;36:292–296. - PubMed
-
- Gregoriadis G, Buckland RA. Enzyme-containing liposomes alleviate a model for storage disease. Nature. 1973;244:170–172. - PubMed
Publication types
MeSH terms
Substances
Grants and funding
- R01 EB012597/EB/NIBIB NIH HHS/United States
- HL092836/HL/NHLBI NIH HHS/United States
- EB012597/EB/NIBIB NIH HHS/United States
- DE019024/DE/NIDCR NIH HHS/United States
- R01 HL092836/HL/NHLBI NIH HHS/United States
- EB008392/EB/NIBIB NIH HHS/United States
- DE021468/DE/NIDCR NIH HHS/United States
- R01 DE021468/DE/NIDCR NIH HHS/United States
- HL099073/HL/NHLBI NIH HHS/United States
- R01 AR057837/AR/NIAMS NIH HHS/United States
- R01 HL099073/HL/NHLBI NIH HHS/United States
- AR057837/AR/NIAMS NIH HHS/United States
- RL1 DE019024/DE/NIDCR NIH HHS/United States
- R01 EB008392/EB/NIBIB NIH HHS/United States
LinkOut - more resources
Full Text Sources
Other Literature Sources