The mechanism of saccade motor pattern generation investigated by a large-scale spiking neuron model of the superior colliculus
- PMID: 23431402
- PMCID: PMC3576366
- DOI: 10.1371/journal.pone.0057134
The mechanism of saccade motor pattern generation investigated by a large-scale spiking neuron model of the superior colliculus
Abstract
The subcortical saccade-generating system consists of the retina, superior colliculus, cerebellum and brainstem motoneuron areas. The superior colliculus is the site of sensory-motor convergence within this basic visuomotor loop preserved throughout the vertebrates. While the system has been extensively studied, there are still several outstanding questions regarding how and where the saccade eye movement profile is generated and the contribution of respective parts within this system. Here we construct a spiking neuron model of the whole intermediate layer of the superior colliculus based on the latest anatomy and physiology data. The model consists of conductance-based spiking neurons with quasi-visual, burst, buildup, local inhibitory, and deep layer inhibitory neurons. The visual input is given from the superficial superior colliculus and the burst neurons send the output to the brainstem oculomotor nuclei. Gating input from the basal ganglia and an integral feedback from the reticular formation are also included.We implement the model in the NEST simulator and show that the activity profile of bursting neurons can be reproduced by a combination of NMDA-type and cholinergic excitatory synaptic inputs and integrative inhibitory feedback. The model shows that the spreading neural activity observed in vivo can keep track of the collicular output over time and reset the system at the end of a saccade through activation of deep layer inhibitory neurons. We identify the model parameters according to neural recording data and show that the resulting model recreates the saccade size-velocity curves known as the saccadic main sequence in behavioral studies. The present model is consistent with theories that the superior colliculus takes a principal role in generating the temporal profiles of saccadic eye movements, rather than just specifying the end points of eye movements.
Conflict of interest statement
Figures
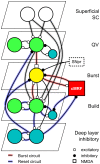
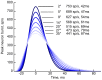
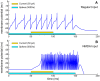
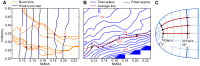



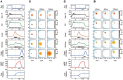




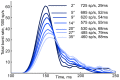
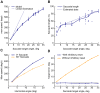



Similar articles
-
Activity of neurons in monkey superior colliculus during interrupted saccades.J Neurophysiol. 1996 Jun;75(6):2562-80. doi: 10.1152/jn.1996.75.6.2562. J Neurophysiol. 1996. PMID: 8793764
-
Activity in deep intermediate layer collicular neurons during interrupted saccades.Exp Brain Res. 2000 Jan;130(2):227-37. doi: 10.1007/s002219900239. Exp Brain Res. 2000. PMID: 10672476
-
Macaque frontal eye field input to saccade-related neurons in the superior colliculus.J Neurophysiol. 2003 Aug;90(2):1046-62. doi: 10.1152/jn.00072.2003. Epub 2003 May 7. J Neurophysiol. 2003. PMID: 12736234
-
Neural control of saccades.Prog Brain Res. 2002;140:21-49. doi: 10.1016/S0079-6123(02)40040-4. Prog Brain Res. 2002. PMID: 12508580 Review.
-
Exploring the superior colliculus in vitro.J Neurophysiol. 2009 Nov;102(5):2581-93. doi: 10.1152/jn.00498.2009. Epub 2009 Aug 26. J Neurophysiol. 2009. PMID: 19710376 Free PMC article. Review.
Cited by
-
Audiovisual Rehabilitation in Hemianopia: A Model-Based Theoretical Investigation.Front Comput Neurosci. 2017 Dec 15;11:113. doi: 10.3389/fncom.2017.00113. eCollection 2017. Front Comput Neurosci. 2017. PMID: 29326578 Free PMC article.
-
A spiking neural network model of the midbrain superior colliculus that generates saccadic motor commands.Biol Cybern. 2017 Aug;111(3-4):249-268. doi: 10.1007/s00422-017-0719-9. Epub 2017 May 20. Biol Cybern. 2017. PMID: 28528360 Free PMC article.
-
On the Cranial Nerves.NeuroSci. 2023 Dec 28;5(1):8-38. doi: 10.3390/neurosci5010002. eCollection 2024 Mar. NeuroSci. 2023. PMID: 39483811 Free PMC article. Review.
-
Integrating Brain and Biomechanical Models-A New Paradigm for Understanding Neuro-muscular Control.Front Neurosci. 2018 Feb 6;12:39. doi: 10.3389/fnins.2018.00039. eCollection 2018. Front Neurosci. 2018. PMID: 29467606 Free PMC article.
-
Limitations of short range Mexican hat connection for driving target selection in a 2D neural field: activity suppression and deviation from input stimuli.Front Comput Neurosci. 2015 Oct 20;9:128. doi: 10.3389/fncom.2015.00128. eCollection 2015. Front Comput Neurosci. 2015. PMID: 26539103 Free PMC article.
References
Publication types
MeSH terms
Grants and funding
LinkOut - more resources
Full Text Sources
Other Literature Sources
Molecular Biology Databases