Spectral identification of lighting type and character
- PMID: 22319336
- PMCID: PMC3274255
- DOI: 10.3390/s100403961
Spectral identification of lighting type and character
Abstract
We investigated the optimal spectral bands for the identification of lighting types and the estimation of four major indices used to measure the efficiency or character of lighting. To accomplish these objectives we collected high-resolution emission spectra (350 to 2,500 nm) for forty-three different lamps, encompassing nine of the major types of lamps used worldwide. The narrow band emission spectra were used to simulate radiances in eight spectral bands including the human eye photoreceptor bands (photopic, scotopic, and "meltopic") plus five spectral bands in the visible and near-infrared modeled on bands flown on the Landsat Thematic Mapper (TM). The high-resolution continuous spectra are superior to the broad band combinations for the identification of lighting type and are the standard for calculation of Luminous Efficacy of Radiation (LER), Correlated Color Temperature (CCT) and Color Rendering Index (CRI). Given the high cost that would be associated with building and flying a hyperspectral sensor with detection limits low enough to observe nighttime lights we conclude that it would be more feasible to fly an instrument with a limited number of broad spectral bands in the visible to near infrared. The best set of broad spectral bands among those tested is blue, green, red and NIR bands modeled on the band set flown on the Landsat Thematic Mapper. This set provides low errors on the identification of lighting types and reasonable estimates of LER and CCT when compared to the other broad band set tested. None of the broad band sets tested could make reasonable estimates of Luminous Efficacy (LE) or CRI. The photopic band proved useful for the estimation of LER. However, the three photoreceptor bands performed poorly in the identification of lighting types when compared to the bands modeled on the Landsat Thematic Mapper. Our conclusion is that it is feasible to identify lighting type and make reasonable estimates of LER and CCT using four or more spectral bands with minimal spectral overlap spanning the 0.4 to 1.0 um region.
Keywords: LED; Nightsat; lighting efficiency; lighting types; nighttime lights; photopic band.
Figures
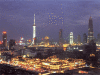
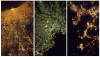
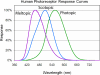
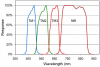
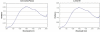
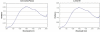
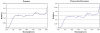
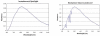
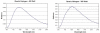
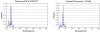
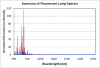
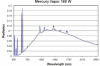
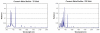
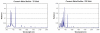
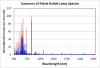
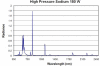
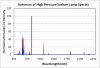
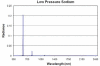
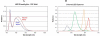
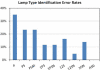
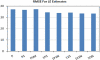
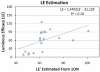
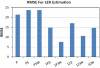
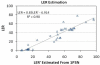
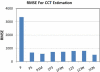
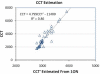
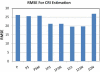
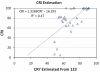
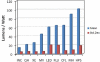
Similar articles
-
Optimized Performance Parameters for Nighttime Multispectral Satellite Imagery to Analyze Lightings in Urban Areas.Sensors (Basel). 2020 Jun 10;20(11):3313. doi: 10.3390/s20113313. Sensors (Basel). 2020. PMID: 32532117 Free PMC article.
-
Limiting the impact of light pollution on human health, environment and stellar visibility.J Environ Manage. 2011 Oct;92(10):2714-22. doi: 10.1016/j.jenvman.2011.06.029. Epub 2011 Jul 13. J Environ Manage. 2011. PMID: 21745709
-
Spectral optimization of phosphor-coated white LED for road lighting based on the mesopic limited luminous efficacy and IES color fidelity index.Appl Opt. 2018 Feb 1;57(4):931-936. doi: 10.1364/AO.57.000931. Appl Opt. 2018. PMID: 29400769
-
Optimizing spectral compositions of multichannel LED light sources by IES color fidelity index and luminous efficacy of radiation.Appl Opt. 2017 Mar 1;56(7):1962-1971. doi: 10.1364/AO.56.001962. Appl Opt. 2017. PMID: 28248396
-
The possible ocular hazards of LED dental illumination applications.J Tenn Dent Assoc. 2013 Fall-Winter;93(2):25-9; quiz 30-1. J Tenn Dent Assoc. 2013. PMID: 24611218 Review.
Cited by
-
The impact of artificial light at night on nocturnal insects: A review and synthesis.Ecol Evol. 2018 Oct 23;8(22):11337-11358. doi: 10.1002/ece3.4557. eCollection 2018 Nov. Ecol Evol. 2018. PMID: 30519447 Free PMC article. Review.
-
Mapping Urban Extent Using Luojia 1-01 Nighttime Light Imagery.Sensors (Basel). 2018 Oct 29;18(11):3665. doi: 10.3390/s18113665. Sensors (Basel). 2018. PMID: 30380616 Free PMC article.
-
Human Activity Changes During COVID-19 Lockdown in China-A View From Nighttime Light.Geohealth. 2022 Aug 1;6(8):e2021GH000555. doi: 10.1029/2021GH000555. eCollection 2022 Aug. Geohealth. 2022. PMID: 35942293 Free PMC article.
-
Mapping lightscapes: spatial patterning of artificial lighting in an urban landscape.PLoS One. 2013 May 6;8(5):e61460. doi: 10.1371/journal.pone.0061460. Print 2013. PLoS One. 2013. PMID: 23671566 Free PMC article.
-
On-Orbit Signal-to-Noise Ratio Test Method for Night-Light Camera in Luojia 1-01 Satellite Based on Time-Sequence Imagery.Sensors (Basel). 2019 Sep 20;19(19):4077. doi: 10.3390/s19194077. Sensors (Basel). 2019. PMID: 31547198 Free PMC article.
References
-
- International Energy Agency . Light’s Labour Lost. Paris, France: 2006.
-
- Mills E. The specter of fuel based lighting. Science. 2005;308:1263–1264. - PubMed
-
- Rich C., Longcore T. Ecological Consequences of Artificial Night Lighting. Island Press; Washington, DC, USA: 2005.
-
- Schubert E.F., Kim J.K. Solid state light sources getting smarter. Science. 2005;308:1274–1278. - PubMed
-
- Croft T.A. Night-time images of the earth from space. Sci. Amer. 1978;239:68–79.
LinkOut - more resources
Full Text Sources
Other Literature Sources
Miscellaneous