Metal oxide gas sensors: sensitivity and influencing factors
- PMID: 22294916
- PMCID: PMC3264469
- DOI: 10.3390/s100302088
Metal oxide gas sensors: sensitivity and influencing factors
Abstract
Conductometric semiconducting metal oxide gas sensors have been widely used and investigated in the detection of gases. Investigations have indicated that the gas sensing process is strongly related to surface reactions, so one of the important parameters of gas sensors, the sensitivity of the metal oxide based materials, will change with the factors influencing the surface reactions, such as chemical components, surface-modification and microstructures of sensing layers, temperature and humidity. In this brief review, attention will be focused on changes of sensitivity of conductometric semiconducting metal oxide gas sensors due to the five factors mentioned above.
Keywords: gas sensors; metal oxide; sensitivity; surface reaction.
Figures
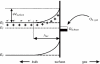
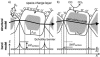
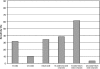
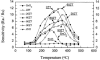
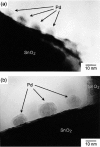
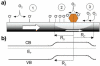
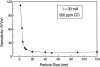
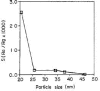
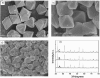
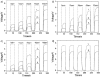
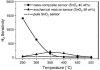
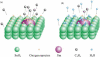
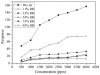
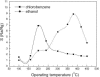
Similar articles
-
Metal oxide nanostructures and their gas sensing properties: a review.Sensors (Basel). 2012;12(3):2610-31. doi: 10.3390/s120302610. Epub 2012 Feb 27. Sensors (Basel). 2012. PMID: 22736968 Free PMC article. Review.
-
Current Understanding of the Fundamental Mechanisms of Doped and Loaded Semiconducting Metal-Oxide-Based Gas Sensing Materials.ACS Sens. 2019 Sep 27;4(9):2228-2249. doi: 10.1021/acssensors.9b00975. Epub 2019 Aug 21. ACS Sens. 2019. PMID: 31365820 Review.
-
Metal oxide semi-conductor gas sensors in environmental monitoring.Sensors (Basel). 2010;10(6):5469-502. doi: 10.3390/s100605469. Epub 2010 Jun 1. Sensors (Basel). 2010. PMID: 22219672 Free PMC article. Review.
-
An Innovative Modular eNose System Based on a Unique Combination of Analog and Digital Metal Oxide Sensors.ACS Sens. 2019 Sep 27;4(9):2277-2281. doi: 10.1021/acssensors.9b01244. Epub 2019 Aug 21. ACS Sens. 2019. PMID: 31389228
-
Surface studies of gas sensing metal oxides.Phys Chem Chem Phys. 2007 May 21;9(19):2307-18. doi: 10.1039/b617710g. Epub 2007 Feb 19. Phys Chem Chem Phys. 2007. PMID: 17492094
Cited by
-
Engineered Electroactive Solutions for Electrochemical Detection of Tuberculosis-Associated Volatile Organic Biomarkers.IEEE Sens J. 2022 Feb 15;22(4):2984-2992. doi: 10.1109/jsen.2021.3126732. Epub 2021 Nov 8. IEEE Sens J. 2022. PMID: 36157103 Free PMC article.
-
Metal oxide nanostructures and their gas sensing properties: a review.Sensors (Basel). 2012;12(3):2610-31. doi: 10.3390/s120302610. Epub 2012 Feb 27. Sensors (Basel). 2012. PMID: 22736968 Free PMC article. Review.
-
A Study of MgZnO Thin Film for Hydrogen Sensing Application.Materials (Basel). 2024 Jul 25;17(15):3677. doi: 10.3390/ma17153677. Materials (Basel). 2024. PMID: 39124342 Free PMC article.
-
Tin Oxide Based Hybrid Nanostructures for Efficient Gas Sensing.Molecules. 2022 Oct 18;27(20):7038. doi: 10.3390/molecules27207038. Molecules. 2022. PMID: 36296632 Free PMC article. Review.
-
Reproducibility and Repeatability Tests on (SnTiNb)O2 Sensors in Detecting ppm-Concentrations of CO and Up to 40% of Humidity: A Statistical Approach.Sensors (Basel). 2023 Feb 10;23(4):1983. doi: 10.3390/s23041983. Sensors (Basel). 2023. PMID: 36850578 Free PMC article.
References
-
- Korotcenkov G. Metal Oxides for Solid-State Gas Sensors: What Determines Our Choice? Mater. Sci. Eng. B. 2007;139:1–23.
-
- Barsan N., Koziej D., Weimar U. Metal Oxide-Based Gas Sensor Research: How to? Sens. Actuat. B. 2007;121:18–35.
-
- Korotcenkov G. The Role of Morphology and Crystallographic Structure of Metal Oxides in Response of Conductometric-Type Gas Sensors. Mater. Sci. Eng R. 2008;61:1–39.
-
- Batzill M. Surface Science Studies of Gas Sensing Materials: SnO2. Sensors. 2006;6:1345–1366.
-
- Barsan N., Schweizer-Berberich M., Göpel W. Fundamental and Practical Aspects in the Design of Nanoscaled SnO2 Gas Sensors: a Status Report. Fresenius J. Anal. Chem. 1999;365:287–304.
Publication types
MeSH terms
Substances
LinkOut - more resources
Full Text Sources
Other Literature Sources