TNF/p38α/polycomb signaling to Pax7 locus in satellite cells links inflammation to the epigenetic control of muscle regeneration
- PMID: 20887952
- PMCID: PMC2951277
- DOI: 10.1016/j.stem.2010.08.013
TNF/p38α/polycomb signaling to Pax7 locus in satellite cells links inflammation to the epigenetic control of muscle regeneration
Abstract
How regeneration cues are converted into the epigenetic information that controls gene expression in adult stem cells is currently unknown. We identified an inflammation-activated signaling in muscle stem (satellite) cells, by which the polycomb repressive complex 2 (PRC2) represses Pax7 expression during muscle regeneration. TNF-activated p38α kinase promotes the interaction between YY1 and PRC2, via threonine 372 phosphorylation of EZH2, the enzymatic subunit of the complex, leading to the formation of repressive chromatin on Pax7 promoter. TNF-α antibodies stimulate satellite cell proliferation in regenerating muscles of dystrophic or normal mice. Genetic knockdown or pharmacological inhibition of the enzymatic components of the p38/PRC2 signaling--p38α and EZH2--invariably promote Pax7 expression and expansion of satellite cells that retain their differentiation potential upon signaling resumption. Genetic knockdown of Pax7 impaired satellite cell proliferation in response to p38 inhibition, thereby establishing the biological link between p38/PRC2 signaling to Pax7 and satellite cell decision to proliferate or differentiate.
Copyright © 2010 Elsevier Inc. All rights reserved.
Figures
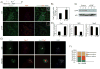
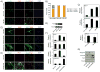
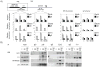
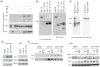
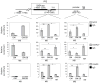
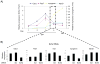
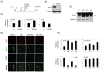
Comment in
-
The yin and yang of polycomb repression in regenerating muscle.Cell Stem Cell. 2010 Oct 8;7(4):422-4. doi: 10.1016/j.stem.2010.09.005. Cell Stem Cell. 2010. PMID: 20887945
Similar articles
-
Selective control of Pax7 expression by TNF-activated p38α/polycomb repressive complex 2 (PRC2) signaling during muscle satellite cell differentiation.Cell Cycle. 2011 Jan 15;10(2):191-8. doi: 10.4161/cc.10.2.14441. Epub 2011 Jan 15. Cell Cycle. 2011. PMID: 21220942
-
HMGB1-RAGE regulates muscle satellite cell homeostasis through p38-MAPK- and myogenin-dependent repression of Pax7 transcription.J Cell Sci. 2012 Mar 15;125(Pt 6):1440-54. doi: 10.1242/jcs.092163. Epub 2012 Feb 10. J Cell Sci. 2012. PMID: 22328527
-
The Ror1 receptor tyrosine kinase plays a critical role in regulating satellite cell proliferation during regeneration of injured muscle.J Biol Chem. 2017 Sep 22;292(38):15939-15951. doi: 10.1074/jbc.M117.785709. Epub 2017 Aug 8. J Biol Chem. 2017. PMID: 28790171 Free PMC article.
-
Concise Review: Epigenetic Regulation of Myogenesis in Health and Disease.Stem Cells Transl Med. 2016 Mar;5(3):282-90. doi: 10.5966/sctm.2015-0266. Epub 2016 Jan 21. Stem Cells Transl Med. 2016. PMID: 26798058 Free PMC article. Review.
-
The molecular regulation of muscle stem cell function.Cold Spring Harb Symp Quant Biol. 2008;73:323-31. doi: 10.1101/sqb.2008.73.064. Epub 2009 Mar 27. Cold Spring Harb Symp Quant Biol. 2008. PMID: 19329572 Review.
Cited by
-
EZH2: a pivotal regulator in controlling cell differentiation.Am J Transl Res. 2012;4(4):364-75. Epub 2012 Oct 10. Am J Transl Res. 2012. PMID: 23145205 Free PMC article.
-
Posttranslational regulation of self-renewal capacity: insights from proteome and phosphoproteome analyses of stem cell leukemia.Blood. 2012 Aug 23;120(8):e17-27. doi: 10.1182/blood-2011-12-397844. Epub 2012 Jul 16. Blood. 2012. PMID: 22802335 Free PMC article.
-
Pharmacological inhibition of EZH2 as a promising differentiation therapy in embryonal RMS.BMC Cancer. 2014 Feb 27;14:139. doi: 10.1186/1471-2407-14-139. BMC Cancer. 2014. PMID: 24575771 Free PMC article.
-
Epigenetic regulation of satellite cell activation during muscle regeneration.Stem Cell Res Ther. 2011 Apr 19;2(2):18. doi: 10.1186/scrt59. Stem Cell Res Ther. 2011. PMID: 21542881 Free PMC article. Review.
-
ZEB1 protects skeletal muscle from damage and is required for its regeneration.Nat Commun. 2019 Mar 25;10(1):1364. doi: 10.1038/s41467-019-08983-8. Nat Commun. 2019. PMID: 30910999 Free PMC article.
References
-
- Bernstein BE, Mikkelsen TS, Xie X, Kamal M, Huebert DJ, Cuff J, Fry B, Meissner A, Wernig M, Plath K, Jaenisch R, Wagschal A, Feil R, Schreiber SL, Lander ES. A bivalent chromatin structure marks key developmental genes in embryonic stem cells. Cell. 2006;125(2):315–26. - PubMed
-
- Boyer LA, Mathur D, Jaenisch R. Molecular control of pluripotency. Curr Opin Genet Dev. 2006a;16:455–462. - PubMed
-
- Boyer LA, Plath K, Zeitlinger J, Brambrink T, Medeiros LA, Lee TI, Levine SS, Wernig M, Tajonar A, Ray MK, Bell GW, Otte AP, Vidal M, Gifford DK, Young RA, Jaenisch R. Polycomb complexes repress developmental regulators in murine embryonic stem cells. Nature. 2006b;7091:349–53. 2006. - PubMed
Publication types
MeSH terms
Substances
Grants and funding
LinkOut - more resources
Full Text Sources
Other Literature Sources
Molecular Biology Databases