Three dysregulated miRNAs control kallikrein 10 expression and cell proliferation in ovarian cancer
- PMID: 20354523
- PMCID: PMC2856011
- DOI: 10.1038/sj.bjc.6605634
Three dysregulated miRNAs control kallikrein 10 expression and cell proliferation in ovarian cancer
Abstract
Background: Kallikrein-related peptidases (KLKs) are a family of serine proteases that have been shown to be dysregulated in several malignancies including ovarian cancer. The control of kallikrein genes and their physiological function in cancer is not well understood. We hypothesized that microRNAs (miRNAs) represent a novel mechanism for post-transcriptional control of KLK expression in cancer.
Methods: We first analysed miRNA expression in ovarian cancer in silico. A total of 98 miRNAs were reported to have altered expression in ovarian cancer. Three of these miRNAs were predicted to target KLK10. We experimentally verified the predicted miR-KLK10 interaction using two independent techniques, a luciferase assay with a construct containing the KLK10 3' untranslated region (UTR), pMIR-KLK10, and measuring KLK10 protein levels after transfection with miRNA.
Results: When we co-transfected cells with pMIR-KLK10 and either let-7f, miR-224, or mR-516a, we saw decreased luciferase signal, suggesting that these miRNAs can target KLK10. We then examined the effect of these three miRNAs on KLK10 protein expression and cell growth. Transfection of all miRNAs, let-7f, miR-224, and miR-516a led to a decrease in protein expression and cellular growth. This effect was shown to be dose dependent. The KLK10 protein levels were partially restored by co-transfecting let-7f and its inhibitor. In addition, there was a slight decrease in KLK10 mRNA expression after transfection with let-7f.
Conclusion: Our results confirm that KLKs can be targeted by more than one miRNA. Increased expression of certain miRNAs in ovarian cancer can lead to decreased KLK protein expression and subsequently have a negative effect on cell proliferation. This dose-dependent effect suggests that a 'tweaking' or 'fine-tuning' mechanism exists in which the expression of one KLK can be controlled by multiple miRNAs. These data together suggest that miRNA may be used as potential therapeutic options and further studies are required.
Figures
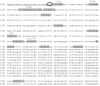
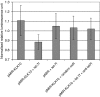
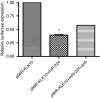
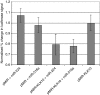
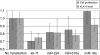
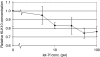
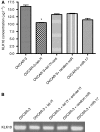
Similar articles
-
Kallikreins as microRNA targets: an in silico and experimental-based analysis.Biol Chem. 2008 Jun;389(6):731-8. doi: 10.1515/BC.2008.071. Biol Chem. 2008. PMID: 18627289
-
Dysregulation of kallikrein-related peptidases in renal cell carcinoma: potential targets of miRNAs.Biol Chem. 2010 Apr;391(4):411-23. doi: 10.1515/BC.2010.041. Biol Chem. 2010. PMID: 20180642
-
The miRNA-kallikrein axis of interaction: a new dimension in the pathogenesis of prostate cancer.Biol Chem. 2012 Apr;393(5):379-89. doi: 10.1515/hsz-2011-0246. Biol Chem. 2012. PMID: 22505520
-
The miRNA-kallikrein interaction: a mosaic of epigenetic regulation in cancer.Biol Chem. 2018 Sep 25;399(9):973-982. doi: 10.1515/hsz-2018-0112. Biol Chem. 2018. PMID: 29604203 Review.
-
Epigenetic regulation of kallikrein-related peptidases: there is a whole new world out there.Biol Chem. 2012 Apr;393(5):319-30. doi: 10.1515/hsz-2011-0273. Biol Chem. 2012. PMID: 22505515 Review.
Cited by
-
Toward an integrative analysis of the tumor microenvironment in ovarian epithelial carcinoma.Cancer Microenviron. 2012 Aug;5(2):173-83. doi: 10.1007/s12307-011-0092-5. Epub 2011 Nov 23. Cancer Microenviron. 2012. PMID: 22109660 Free PMC article.
-
Chemoprevention activity of dipyridamole in the MMTV-PyMT transgenic mouse model of breast cancer.Cancer Prev Res (Phila). 2013 May;6(5):437-47. doi: 10.1158/1940-6207.CAPR-12-0345. Epub 2013 Feb 27. Cancer Prev Res (Phila). 2013. PMID: 23447563 Free PMC article.
-
miR-6884-5p inhibits proliferation and epithelial-mesenchymal transition in non-small cell lung cancer cells.Heliyon. 2024 Sep 25;10(19):e38428. doi: 10.1016/j.heliyon.2024.e38428. eCollection 2024 Oct 15. Heliyon. 2024. PMID: 39391483 Free PMC article.
-
miRNA profiling in metastatic renal cell carcinoma reveals a tumour-suppressor effect for miR-215.Br J Cancer. 2011 Nov 22;105(11):1741-9. doi: 10.1038/bjc.2011.401. Epub 2011 Oct 27. Br J Cancer. 2011. PMID: 22033272 Free PMC article.
-
Epigenetic impacts of ascorbate on human metastatic melanoma cells.Front Oncol. 2014 Aug 25;4:227. doi: 10.3389/fonc.2014.00227. eCollection 2014. Front Oncol. 2014. PMID: 25202679 Free PMC article.
References
-
- Bando Y, Ito S, Nagai Y, Terayama R, Kishibe M, Jiang YP, Mitrovic B, Takahashi T, Yoshida S (2006) Implications of protease M/neurosin in myelination during experimental demyelination and remyelination. Neurosci Lett 405: 175–180 - PubMed
-
- Baudis M, Cleary ML (2001) Progenetix.net: an online repository for molecular cytogenetic aberration data. Bioinformatics 17: 1228–1229 - PubMed
Publication types
MeSH terms
Substances
Grants and funding
LinkOut - more resources
Full Text Sources