SOCS3 deletion promotes optic nerve regeneration in vivo
- PMID: 20005819
- PMCID: PMC2796263
- DOI: 10.1016/j.neuron.2009.11.021
SOCS3 deletion promotes optic nerve regeneration in vivo
Abstract
Axon regeneration failure accounts for permanent functional deficits following CNS injury in adult mammals. However, the underlying mechanisms remain elusive. In analyzing axon regeneration in different mutant mouse lines, we discovered that deletion of suppressor of cytokine signaling 3 (SOCS3) in adult retinal ganglion cells (RGCs) promotes robust regeneration of injured optic nerve axons. This regeneration-promoting effect is efficiently blocked in SOCS3-gp130 double-knockout mice, suggesting that SOCS3 deletion promotes axon regeneration via a gp130-dependent pathway. Consistently, a transient upregulation of ciliary neurotrophic factor (CNTF) was observed within the retina following optic nerve injury. Intravitreal application of CNTF further enhances axon regeneration from SOCS3-deleted RGCs. Together, our results suggest that compromised responsiveness to injury-induced growth factors in mature neurons contributes significantly to regeneration failure. Thus, developing strategies to modulate negative signaling regulators may be an efficient strategy of promoting axon regeneration after CNS injury.
Figures
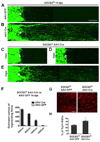
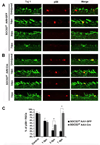
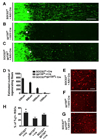
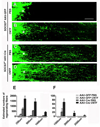
Comment in
-
Taking off the SOCS: cytokine signaling spurs regeneration.Neuron. 2009 Dec 10;64(5):591-2. doi: 10.1016/j.neuron.2009.11.027. Neuron. 2009. PMID: 20005813
Similar articles
-
Negative impact of rAAV2 mediated expression of SOCS3 on the regeneration of adult retinal ganglion cell axons.Mol Cell Neurosci. 2011 Feb;46(2):507-15. doi: 10.1016/j.mcn.2010.12.003. Epub 2010 Dec 9. Mol Cell Neurosci. 2011. PMID: 21145973
-
Impact of PTEN/SOCS3 deletion on amelioration of dendritic shrinkage of retinal ganglion cells after optic nerve injury.Exp Eye Res. 2020 Mar;192:107938. doi: 10.1016/j.exer.2020.107938. Epub 2020 Jan 21. Exp Eye Res. 2020. PMID: 31972211
-
Three-dimensional evaluation of retinal ganglion cell axon regeneration and pathfinding in whole mouse tissue after injury.Exp Neurol. 2013 Sep;247:653-62. doi: 10.1016/j.expneurol.2013.03.001. Epub 2013 Mar 16. Exp Neurol. 2013. PMID: 23510761 Free PMC article.
-
Hyper-IL-6: a potent and efficacious stimulator of RGC regeneration.Eye (Lond). 2017 Feb;31(2):173-178. doi: 10.1038/eye.2016.234. Epub 2016 Nov 25. Eye (Lond). 2017. PMID: 27886185 Free PMC article. Review.
-
Lost in the jungle: new hurdles for optic nerve axon regeneration.Trends Neurosci. 2014 Jul;37(7):381-7. doi: 10.1016/j.tins.2014.05.002. Epub 2014 May 26. Trends Neurosci. 2014. PMID: 24874558 Review.
Cited by
-
Siponimod exerts neuroprotective effects on the retina and higher visual pathway through neuronal S1PR1 in experimental glaucoma.Neural Regen Res. 2023 Apr;18(4):840-848. doi: 10.4103/1673-5374.344952. Neural Regen Res. 2023. PMID: 36204852 Free PMC article.
-
Subtype-specific regeneration of retinal ganglion cells following axotomy: effects of osteopontin and mTOR signaling.Neuron. 2015 Mar 18;85(6):1244-56. doi: 10.1016/j.neuron.2015.02.017. Epub 2015 Mar 5. Neuron. 2015. PMID: 25754821 Free PMC article.
-
Targeting PTEN but not SOCS3 resists an age-dependent decline in promoting axon sprouting.iScience. 2022 Oct 17;25(11):105383. doi: 10.1016/j.isci.2022.105383. eCollection 2022 Nov 18. iScience. 2022. PMID: 36339257 Free PMC article.
-
Loss of Arid1a Promotes Neuronal Survival Following Optic Nerve Injury.Front Cell Neurosci. 2020 May 15;14:131. doi: 10.3389/fncel.2020.00131. eCollection 2020. Front Cell Neurosci. 2020. PMID: 32670021 Free PMC article.
-
Neuronal Nogo-A upregulation does not contribute to ER stress-associated apoptosis but participates in the regenerative response in the axotomized adult retina.Cell Death Differ. 2012 Jul;19(7):1096-108. doi: 10.1038/cdd.2011.191. Epub 2011 Dec 23. Cell Death Differ. 2012. PMID: 22193546 Free PMC article.
References
-
- Cacalano NA, Sanden D, Johnston JA. Tyrosine-phosphorylated SOCS-3 inhibits STAT activation but binds to p120 RasGAP and activates Ras. Nat Cell Biol. 2001;3:460–465. - PubMed
Publication types
MeSH terms
Substances
Grants and funding
LinkOut - more resources
Full Text Sources
Other Literature Sources
Medical
Molecular Biology Databases