Embodied information processing: vibrissa mechanics and texture features shape micromotions in actively sensing rats
- PMID: 18304488
- PMCID: PMC4391974
- DOI: 10.1016/j.neuron.2007.12.024
Embodied information processing: vibrissa mechanics and texture features shape micromotions in actively sensing rats
Abstract
Peripheral sensory organs provide the first transformation of sensory information, and understanding how their physical embodiment shapes transduction is central to understanding perception. We report the characterization of surface transduction during active sensing in the rodent vibrissa sensory system, a widely used model. Employing high-speed videography, we tracked vibrissae while rats sampled rough and smooth textures. Variation in vibrissa length predicted motion mean frequencies, including for the highest velocity events, indicating that biomechanics, such as vibrissa resonance, shape signals most likely to drive neural activity. Rough surface contact generated large amplitude, high-velocity "stick-slip-ring" events, while smooth surfaces generated smaller and more regular stick-slip oscillations. Both surfaces produced velocities exceeding those applied in reduced preparations, indicating active sensation of surfaces generates more robust drive than previously predicted. These findings demonstrate a key role for embodiment in vibrissal sensing and the importance of input transformations in sensory representation.
Figures
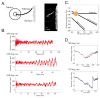
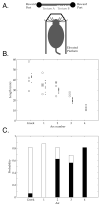
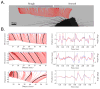
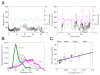
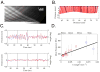
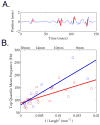
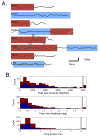
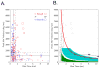
Comment in
-
Response to: Ritt et al., "embodied information processing: vibrissa mechanics and texture features shape micromotions in actively sensing rats." Neuron 57, 599-613.Neuron. 2008 Dec 10;60(5):743-4; author reply 745-7. doi: 10.1016/j.neuron.2008.11.021. Neuron. 2008. PMID: 19081370 No abstract available.
Similar articles
-
Response to: Ritt et al., "embodied information processing: vibrissa mechanics and texture features shape micromotions in actively sensing rats." Neuron 57, 599-613.Neuron. 2008 Dec 10;60(5):743-4; author reply 745-7. doi: 10.1016/j.neuron.2008.11.021. Neuron. 2008. PMID: 19081370 No abstract available.
-
Mechanical resonance enhances the sensitivity of the vibrissa sensory system to near-threshold stimuli.Brain Res. 2008 Oct 15;1235:74-81. doi: 10.1016/j.brainres.2008.06.054. Epub 2008 Jun 24. Brain Res. 2008. PMID: 18625209 Free PMC article.
-
Texture discrimination and multi-unit recording in the rat vibrissal nerve.BMC Neurosci. 2006 May 23;7:42. doi: 10.1186/1471-2202-7-42. BMC Neurosci. 2006. PMID: 16719904 Free PMC article.
-
Frequency-dependent processing in the vibrissa sensory system.J Neurophysiol. 2004 Jun;91(6):2390-9. doi: 10.1152/jn.00925.2003. J Neurophysiol. 2004. PMID: 15136599 Review.
-
A night in the life of a rat: vibrissal mechanics and tactile exploration.Ann N Y Acad Sci. 2011 Apr;1225:110-8. doi: 10.1111/j.1749-6632.2011.06007.x. Ann N Y Acad Sci. 2011. PMID: 21534998 Review.
Cited by
-
Temporally Local Tactile Codes Can Be Stored in Working Memory.Front Hum Neurosci. 2022 May 27;16:840108. doi: 10.3389/fnhum.2022.840108. eCollection 2022. Front Hum Neurosci. 2022. PMID: 35712533 Free PMC article.
-
Spatiotemporal Patterns of Contact Across the Rat Vibrissal Array During Exploratory Behavior.Front Behav Neurosci. 2016 Jan 5;9:356. doi: 10.3389/fnbeh.2015.00356. eCollection 2015. Front Behav Neurosci. 2016. PMID: 26778990 Free PMC article.
-
Support for the slip hypothesis from whisker-related tactile perception of rats in a noisy environment.Front Integr Neurosci. 2015 Oct 15;9:53. doi: 10.3389/fnint.2015.00053. eCollection 2015. Front Integr Neurosci. 2015. PMID: 26528148 Free PMC article.
-
Dynamic correlation between whisking and breathing rhythms in mice.J Neurosci. 2012 Feb 1;32(5):1653-9. doi: 10.1523/JNEUROSCI.4395-11.2012. J Neurosci. 2012. PMID: 22302807 Free PMC article.
-
Sensory cortex underpinnings of traumatic brain injury deficits.PLoS One. 2012;7(12):e52169. doi: 10.1371/journal.pone.0052169. Epub 2012 Dec 21. PLoS One. 2012. PMID: 23284921 Free PMC article.
References
-
- von Bekesy G. Experiments in hearing. Vol. 745. New York: McGraw Hill; 1960.
-
- Geisler C. From sound to synapse. New York: Oxford University Press; 1998.
-
- Woolsey TA, Van der Loos H. The structural organization of layer IV in the somatosensory region (SI) of mouse cerebral cortex. The description of a cortical field composed of discrete cytoarchitectonic units. Brain Res. 1970;17(2):205–42. - PubMed
-
- Jacobs GH, Fenwick JA, Williams GA. Cone-based vision of rats for ultraviolet and visible lights. J Exp Biol. 2001;204(Pt 14):2439–46. - PubMed
-
- Pinto DJ, Brumberg JC, Simons DJ. Circuit dynamics and coding strategies in rodent somatosensory cortex. J Neurophysiol. 2000;83(3):1158–66. - PubMed
Publication types
MeSH terms
Grants and funding
LinkOut - more resources
Full Text Sources
Other Literature Sources